Carbohydrate metabolism and fructose metabolism -
Fatty acid degradation Beta oxidation Fatty acid synthesis. Steroid metabolism Sphingolipid metabolism Eicosanoid metabolism Ketosis Reverse cholesterol transport. Metal metabolism Iron metabolism Ethanol metabolism Phospagen system ATP-PCr. Metabolism map. Carbon fixation.
Photo- respiration. Pentose phosphate pathway. Citric acid cycle. Glyoxylate cycle. Urea cycle. Fatty acid synthesis. Fatty acid elongation. Beta oxidation. beta oxidation. Glyco- genolysis.
Glyco- genesis. Glyco- lysis. Gluconeo- genesis. Pyruvate decarb- oxylation. Keto- lysis. Keto- genesis. feeders to gluconeo- genesis. Light reaction. Oxidative phosphorylation. Amino acid deamination. Citrate shuttle. MVA pathway. MEP pathway. Shikimate pathway.
Glycosyl- ation. Sugar acids. Simple sugars. Nucleotide sugars. Propionyl -CoA. Acetyl -CoA. Oxalo- acetate. Succinyl -CoA. α-Keto- glutarate. Ketone bodies. Respiratory chain. Serine group. Branched-chain amino acids. Aspartate group. Amino acids. Ascorbate vitamin C. Bile pigments.
Cobalamins vitamin B Various vitamin Bs. Calciferols vitamin D. Retinoids vitamin A. Nucleic acids. Terpenoid backbones. Bile acids. Glycero- phospholipids. Fatty acids. Glyco- sphingolipids. Polyunsaturated fatty acids. Endo- cannabinoids. Fructose-bisphosphate aldolase Aldolase A , B , C Triosephosphate isomerase.
Glyceraldehyde 3-phosphate dehydrogenase Phosphoglycerate kinase Phosphoglycerate mutase Enolase Pyruvate kinase PKLR , PKM2. Pyruvate carboxylase Phosphoenolpyruvate carboxykinase.
Lactate dehydrogenase. Alanine transaminase. Glycerol kinase Glycerol dehydrogenase. Fructose 6-P,2-kinase:fructose 2,6-bisphosphatase PFKFB1 , PFKFB2 , PFKFB3 , PFKFB4 Bisphosphoglycerate mutase. Metabolism : carbohydrate metabolism , glycogenesis and glycogenolysis enzymes.
Phosphoglucomutase UDP-glucose pyrophosphorylase Glycogen synthase Glycogen branching enzyme Glycogenin. Glycogen phosphorylase Debranching enzyme Phosphoglucomutase.
Alpha-glucosidase Acid. Phosphorylase kinase Protein phosphatase. Metabolism : carbohydrate metabolism fructose and galactose enzymes. Hepatic fructokinase Aldolase B Triokinase.
Sorbitol dehydrogenase Aldose reductase. Lactose synthase Lactase. Mannose phosphate isomerase. Metabolism : carbohydrate metabolism · pentose phosphate pathway enzymes. Glucosephosphate dehydrogenase 6-phosphogluconolactonase Phosphogluconate dehydrogenase.
Phosphopentose isomerase Phosphopentose epimerase Transketolase Transaldolase. Metabolism : carbohydrate metabolism proteoglycan enzymes. L-xylulose reductase L-gulonolactone oxidase UDP-glucuronate 5'-epimerase Xylosyltransferase Sulfotransferase Heparan sulfate EXT1 EXT2 Chondroitin sulfate PAPSS1 PAPSS2.
Iduronatesulfatase Iduronidase. Heparan sulfamidase N-acetyltransferase Alpha-N-acetylglucosaminidase Glucuronidase N-acetylglucosaminesulfatase. Arylsulfatase B Galactosamine-6 sulfatase Beta-galactosidase GLB1.
Metabolism : carbohydrate metabolism · glycoprotein enzymes. Dolichol kinase GCS1 Oligosaccharyltransferase. Neuraminidase Beta-galactosidase Hexosaminidase mannosidase alpha-Mannosidase beta-mannosidase Aspartylglucosaminidase Fucosidase NAGA. N-acetylglucosaminephosphate transferase.
Authority control databases : National Czech Republic. Category : Metabolism. Hidden categories: Articles with short description Short description is different from Wikidata Articles with NKC identifiers. Toggle limited content width. Electron acceptors other than oxygen.
Fatty acid metabolism Fatty acid degradation Beta oxidation Fatty acid synthesis. to oxaloacetate : Pyruvate carboxylase Phosphoenolpyruvate carboxykinase.
extralysosomal: Glycogen phosphorylase Debranching enzyme Phosphoglucomutase. Hunter , Hurler Iduronatesulfatase Iduronidase. Czech Republic. Fructose in muscles and adipose tissue is phosphorylated by hexokinase.
Although the metabolism of fructose and glucose share many of the same intermediate structures, they have very different metabolic fates in human metabolism. Fructose is metabolized almost completely in the liver in humans, and is directed toward replenishment of liver glycogen and triglyceride synthesis, while much of dietary glucose passes through the liver and goes to skeletal muscle, where it is metabolized to CO 2 , H 2 O and ATP , and to fat cells where it is metabolized primarily to glycerol phosphate for triglyceride synthesis as well as energy production.
Hexokinase IV Glucokinase , also occurs in the liver and would be capable of phosphorylating fructose to fructose 6-phosphate an intermediate in the gluconeogenic pathway ; however, it has a relatively high Km 12 mM for fructose and, therefore, essentially all of the fructose is converted to fructosephosphate in the human liver.
Fructosephosphate then undergoes hydrolysis by fructosephosphate aldolase aldolase B to form dihydroxyacetone phosphate DHAP and glyceraldehyde; DHAP can either be isomerized to glyceraldehyde 3-phosphate by triosephosphate isomerase or undergo reduction to glycerol 3-phosphate by glycerol 3-phosphate dehydrogenase.
The glyceraldehyde produced may also be converted to glyceraldehyde 3-phosphate by glyceraldehyde kinase or converted to glycerol 3-phosphate by glyceraldehyde 3-phosphate dehydrogenase. The metabolism of fructose at this point yields intermediates in gluconeogenic pathway leading to glycogen synthesis, or can be oxidized to pyruvate and reduced to lactate, or be decarboxylated to acetyl CoA in the mitochondria and directed toward the synthesis of free fatty acid, resulting finally in triglyceride synthesis.
The synthesis of glycogen in the liver following a fructose-containing meal proceeds from gluconeogenic precursors. Fructose is initially converted to DHAP and glyceraldehyde by fructokinase and aldolase B. The resultant glyceraldehyde then undergoes phosphorylation to glyceraldehydephosphate.
Increased concentrations of DHAP and glyceraldehydephosphate in the liver drive the gluconeogenic pathway toward glucosephosphate, glucosephosphate and glycogen formation. It appears that fructose is a better substrate for glycogen synthesis than glucose and that glycogen replenishment takes precedence over triglyceride formation.
Carbons from dietary fructose are found in both the FFA and glycerol moieties of plasma triglycerides TG. Excess dietary fructose can be converted to pyruvate, enter the Krebs cycle and emerges as citrate directed toward free fatty acid synthesis in the cytosol of hepatocytes.
The DHAP formed during fructolysis can also be converted to glycerol and then glycerol 3-phosphate for TG synthesis. Thus, fructose can provide trioses for both the glycerol 3-phosphate backbone, as well as the free fatty acids in TG synthesis.
Indeed, fructose may provide the bulk of the carbohydrate directed toward de novo TG synthesis in humans. Although not a consistent finding among metabolic feeding studies, diets high in refined fructose have been shown to lead to hypertriglyceridemia in a wide range of populations including individuals with normal glucose metabolism as well as individuals with impaired glucose tolerance, diabetes, hypertriglyceridemia, and hypertension.
The hypertriglyceridemic effects observed are a hallmark of increased dietary carbohydrate, and fructose appears to be dependent on a number of factors including the amount of dietary fructose consumed and degree of insulin resistance.
The lack of two important enzymes in fructose metabolism results in the development of two inborn errors in carbohydrate metabolism — essential fructosuria and hereditary fructose intolerance. In addition, reduced phosphorylation potential within hepatocytes can occur with intravenous infusion of fructose.
The absence of fructokinase results in the inability to phosphorylate fructose to fructosephosphate within the cell. As a result, fructose is neither trapped within the cell nor directed toward its metabolism. This results in an increase in plasma concentration of fructose, eventually exceeding the kidneys' threshold for fructose reabsorption resulting in the appearance of fructose in the urine.
The absence of fructosephosphate aldolase aldolase B results in the accumulation of fructose 1 phosphate in hepatocytes, kidney and small intestines.
An accumulation of fructosephosphate following fructose ingestion inhibits glycogenolysis breakdown of glycogen and gluconeogenesis, resulting in severe hypoglycemia. The incidence varies throughout the world, but it is estimated at , range , to , live births.
Intravenous i. infusion of fructose has been shown to lower phosphorylation potential in liver cells by trapping inorganic phosphate Pi as fructose 1-phosphate.
On the other hand, the splitting of fructose 1 phosphate to DHAP and glyceraldehyde by Aldolase B is relatively slow. Therefore, fructosephosphate accumulates with the corresponding reduction of intracellular Pi available for phosphorylation reactions in the cell.
This is why fructose is contraindicated for total parenteral nutrition TPN solutions and is never given intravenously as a source of carbohydrate. It has been suggested that excessive dietary intake of fructose may also result in reduced phosphorylation potential.
However, this is still a contentious issue. Dietary fructose is not well absorbed and increased dietary intake often results in malabsorption.
Whether or not sufficient amounts of dietary fructose could be absorbed to cause a significant reduction in phosphorylating potential in liver cells remains questionable and there are no clear examples of this in the literature.
Contents move to sidebar hide. Article Talk. Read Edit View history. Tools Tools. What links here Related changes Upload file Special pages Permanent link Page information Cite this page Get shortened URL Download QR code Wikidata item. Download as PDF Printable version.
In other projects. Wikimedia Commons. This article has multiple issues. Please help improve it or discuss these issues on the talk page.
Learn how and when to remove these template messages. This article needs additional citations for verification. Please help improve this article by adding citations to reliable sources. Unsourced material may be challenged and removed.
Find sources: "Fructolysis" — news · newspapers · books · scholar · JSTOR August Learn how and when to remove this template message. This article possibly contains original research. Please improve it by verifying the claims made and adding inline citations.
Statements consisting only of original research should be removed. August Learn how and when to remove this template message. Main article: Essential fructosuria.
Main article: Hereditary fructose intolerance. Carbohydrate Metabolism: synthesis and Oxidation. Missouri: Saunders, Elsevier. doi : ISSN PMC PMID Adv Nutr. Biochemistry 5th ed. ISBN OCLC ISSN X. AJP: Endocrinology and Metabolism.
Skeletal Muscle Metabolism in Exercise and Diabetes. Advances in Experimental Medicine and Biology. Biochemical Journal.
Robin Subcellular Biochemistry: Biochemistry and Biochemical Cell Biology. Sugars and sweeteners. Boca Raton: CRC Press. ISBN X. In Saudubray J, van den Berghe G, Walter JH eds. Inborn Metabolic Diseases: Diagnosis and Treatment 5th ed.
New York: Springer. Retrieved 11 April National Organization for Rare Disorders. Retrieved 1 November
Carbohydrates Carbohydrate metabolism and fructose metabolism mftabolism molecules composed metaabolism carbon, hydrogen, and oxygen frjctose. The family of carbohydrates includes both Fat metabolism science and complex sugars. Glucose and fructose are examples of simple sugars, and starch, glycogen, and cellulose are all examples of complex sugars. The complex sugars are also called polysaccharides and are made of multiple monosaccharide molecules. Polysaccharides serve as energy storage e. Carbohydrate metabolism is the whole of the biochemical processes responsible for the metabolic formationFrutcoseand interconversion of carbohydrates in living organisms. Carbohydrates are Carbohydrafe Carbohydrate metabolism and fructose metabolism many essential metabolic metaboliwm. Humans Energy metabolism and hydration consume a variety of fructoze, digestion breaks down complex carbohydrates into simple monomers monosaccharides : glucosefructosemannose and galactose. After resorption in the gutthe monosaccharides are transported, through the portal veinto the liver, where all non-glucose monosacharids fructose, galactose are transformed into glucose as well. Glycolysis is the process of breaking down a glucose molecule into two pyruvate molecules, while storing energy released during this process as adenosine triphosphate ATP and nicotinamide adenine dinucleotide NADH. Glycolysis consists of ten steps, split into two phases.Meatbolism details. CCarbohydrate dietary fructose as sucrose metaolism high fructose fructse syrup metablism unique effects separate from its role as carbohydrate, or, in fact, Carbohydrat it can be considered Carbohydratee harmful, even a mwtabolism, has assumed prominence metabloism nutrition.
Functional fitness training of the popular and gructose media have already Carbohydrate metabolism and fructose metabolism against fructose and metaboliem for regulation Insulin and gestational diabetes taxation Carbbohydrate from many quarters.
There metaholism conflicting data, however. Outcomes attributed to fructose — obesity, high triglycerides and other features of metabolic syndrome — Carbohydrafe not found in every experimental test and may be more reliably caused by increased total carbohydrate.
Metabolims this review, we try Carbohydeate put fructose in metaboolism by looking metablism the basic metabolic reactions. We conclude that fuctose is best understood as part anc carbohydrate Carbohydrate metabolism and fructose metabolism.
The pathways of fructose and metaboism metabolism Supercharge your performance at the level metaoblism the triose-phosphates frjctose, therefore, any downstream annd also occur with glucose.
In addition, a substantial fructode of ingested fructose is turned to glucose. Regulation of fructose metabolism per Carbohydfateis at the level of substrate control — the lower K m of metabplism compared metbolism glucokinase will metsbolism the Carbohydrate metabolism and fructose metabolism of triose-phosphates.
Generally metaboliam effects of administering fructose alone suggest that fructose metabolism is normally controlled in Csrbohydrate by fructosw. Because the mechanisms of metabokism effects are largely those frcutose a carbohydrate, metaoblism has to ask what the fructpse control should be for experiments that compare ahd to glucose.
In fact, feuctose is metavolism large literature showing benefits in Carbohhydrate total dructose with other nutrients, usually fat, and wnd experiments sensibly constitute the proper control for comparisons of the two sugars. In terms of public health, a Carbohydratee to judgement ketabolism to the Diabetic nephropathy resources story, is likely Carbohydrate metabolism and fructose metabolism Carbohhdrate unpredictable Carbohydrwte and unintended Cabrohydrate.
Popular opinion cannot be ketabolism in metsbolism problem and comparing fructose to ethanol, for example, is without metabolismm correlates.
Carbohysrate, nothing in the biochemistry suggests that Muscle definition exercises for abs is a toxin. Dietary carbohydrate restriction remains the best strategy for obesity, diabetes metaboliam metabolic syndrome. The metaholism contribution of the removal of metabolims or Carbohydrafe to this effect remains unknown.
Fructose as sucrose or high Carbogydrate corn syrup HFCS has become an obsession in nutrition. Painted as Carbohydrate metabolism and fructose metabolism harmful [ 1 ], even a toxin, in mrtabolism popular [ 2 Carbohydrate metabolism and fructose metabolism and scientific publications [ 3 Air displacement plethysmography system 5 ], there are calls for regulation and taxation from many quarters.
While there metabokism little mdtabolism about the benefits of reducing sugar as a metabolisj of restricting caloric frkctose or of reducing total carbohydrates, especially for children, Carrbohydrate studies directly test the effect of metaabolism reduction. Metaboism unique effects fructosse adding fructose compared to glucose are less metabolusm e.
In our opinion, the discussion of fructose shows a lack of restraint that abd reminiscent of what is now recognized mwtabolism a rush Coenzyme Q and exercise judgment on dietary fat and cholesterol.
It ajd worthwhile to gructose back and put fructose frudtose perspective. From an Hormone balance and aging standpoint, fructose, like metsbolism carbohydrates, was a Carbohydrzte of good times.
It is metaabolism believed that the background availability of carbohydrates metabolisk fructose Carbohycrate particular, was low and intermittent in paleolithic ,etabolism.
Fluctuations in dietary scarcity and abundance metabolims likely much greater than they are now. Selective metanolism lay in dealing with the lean Carobhydrate, metabolizing whatever low background fructose was available, qnd well as metwbolism able to handle the high frutcose of consumption that frequently attend sudden good luck.
It is fructos that Carbohyrate might be mtabolism over-consumption metabklism was part of our behavioral frucctose early on and advantage lay in accumulating food adn, as Carbohydrate metabolism and fructose metabolism and glycogen, that might not be Herbal fat metabolism blend in fryctose immediate future.
It Carboydrate important meetabolism remember that evolution is value-free Healthy alternatives to satisfy cravings being able to fruchose a Carbohydrate metabolism and fructose metabolism furctose one sitting might be fructoxe in some circumstances.
In general, what Carbohjdrate called pathology is a human Carbohydfate and not a true metaboism concept; biological systems survive Carbohyfrate adapting to different andd.
In this review, we try to describe how human metabolism metabllism with high fructose input. Eating beyond immediate needs, for any macronutrient, predicts storage in the form ffructose fat or glycogen and high fructose metabolisk is associated with both. ACrbohydrate synthesis, de Cxrbohydrate lipogenesis DNL and TAG synthesis are, in fact, characteristic of high fructose input.
It is in the context of an intermittent and highly reinforcing food source, not the context of a poison, that fructose should be considered. The metabolism of fructose is closely tied to that of glucose Reviews: [ 7 — 10 ]. In this communication, we try to address the following questions: How can unique effects of fructose, increased TAG and insulin resistance, when they are observed, be reconciled with the continuity of glucose and fructose metabolic pathways?
What accounts for specific effects of fructose administered parenterally? Insofar as fructose and glucose are tied to similar pathways, what is the proper control for an experiment in which one sugar is substituted for the other? Our conclusion is that fructose is best understood as part of the general pathways of carbohydrate metabolism.
Any unique effects of fructose are mediated by interactions with glucose as well as by a significant conversion of fructose to glucose. The important difference in the processing of the two sugars lies in the early steps, the much greater affinity of fructokinase compared to glucokinase, that is, regulation is at the level of substrate control, affecting the population of the triose-phosphates.
Because of their close connection, replacing dietary fructose with glucose as a therapeutic measure is expected to be variable, dependent on the particular conditions and individual responses. Even those studies that show unique effects tend to have large errors and many cannot distinguish between the effects of added fructose vs glucose.
In addition, some experimental studies, probably most, require fairly high levels of fructose. Replacing fructose with glucose is also expected to be less effective in reducing risk factors than substitution of fat for any carbohydrate.
Dietary carbohydrate restriction remains the most effective, if under-utilized strategy against obesity, diabetes and metabolic syndrome in trials of various duration and protocols [ 11 — 18 ] and Additional file 1.
From a public health standpoint, the scarcity of studies showing significant improvement of metabolic abnormalities by specific reduction in dietary sucrose or fructose is of concern since that is being recommended for the population at large.
It might be prudent to avoid another grand experiment on the whole population — as the diet-heart paradigm has been described — in the absence of data from even small studies and without consideration of unintended consequences.
Fructose is a carbohydrate. It is processed by incorporation into carbohydrate metabolism. Specific effects that are brought about by fructose elevation derive from an increase in the intermediates of carbohydrate metabolism.
For humans, there is a background of dietary intake in which glucose almost always exceeds fructose and where fructose is rapidly cleared while glucose is maintained at constant levels.
Most important, a significant amount of ingested fructose is converted to glucose Figure 1. Overview of the major aspects of hepatic metabolism of glucose and fructose.
Key points emphasized in the text: the two sugars converge at the level of the triose-phosphates dihydroxyacetone-phosphate DHAP and glyceraldehydeP GaP. Conversion of fructose to the triose-phosphates is unidirectional but fructosephosphate is a positive effector of glucokinase and regulates glycogen synthesis by activating the synthase and inhibiting phosphorylase.
The last effect may be different between species. Light blue arrows show the path of gluconeogenesis from fructose leading to glucoseP which, in turn, can produce glucose or be incorporated into glycogen.
Fructose and glucose metabolism converge at the level of the triose-phosphates Figure 1. The major concerns in fructose metabolism — synthesis of glycerolphosphate glycerolP for triglyceride synthesis, generation of acetyl-CoA for the TCA cycle and de novo lipogenesis DNL — derive from these intermediates.
The conundrum for understanding fructose metabolism is how a carbon in the triose-phosphates knows whether it came from fructose or glucose.
Finally, glucose is the major secretagogue of insulin which controls glycogen metabolism, triglyceride assembly and breakdown, and DNL.
In such a complex system, experimental details become important. In particular, the effect of adding fructose vs glucose to a diet whose background composition is already high in carbohydrate is sensibly different from one where there is low total carbohydrate.
Specific effects of fructose likely represent kinetic effects — more rapid population of intermediates from fructose — but these may also depend on individual conditions.
In a hypercaloric diet, as blood glucose exceeds the high K m of glucokinase, the effects of added glucose or fructose will be indistinguishable. McDevitt, et al. Comparisons of low fructose and glucose are not well studied, presumably because they are not considered to involve a health risk.
Metabolism is value-free, that is, the response to overconsumption of fructose may have been advantageous for an organism with intermittent supply of carbohydrate.
The current discussion in nutrition, however, is couched in terms of good and bad. Under these conditions, any added fructose may be worse than glucose but this is expected to be a minor perturbation in the effect of the high carbohydrate that has already caused, e.
To some extent, the question is a logical one. Numerous studies have demonstrated the benefits of restriction of carbohydrates in general Supplement.
The question, then, is what is the appropriate control for those experiments in which different carbohydrates are compared. If there is a null hypothesis, it is that the effects of fructose are primarily due to its role as a carbohydrate.
The appropriate comparison, then, would be experiments in which carbohydrates across the board are substituted with another macronutrient, generally fat. We will provide an example of the effects of studies in the lab of Jeff Volek that can be compared to fructose studies [ 121424 ].
The liver is not the only organ that metabolizes fructose but hepatic metabolism accounts for at least half of the total and represents the focus of most concern. As a kind of command center in metabolism, the liver distributes energy to other cells in the form of glucose, lactate and triglycerides although other tissues, intestines, kidney and muscle, can also process fructose directly.
In the case of the kidney, lactate and glucose from fructose may also be exported. Glucokinase, the hepatic hexokinase, has a high K m — in the range of normal blood glucose — so that liver and blood glucose are in equilibrium.
Hepatic glucose will fluctuate according to high blood glucose and other acute changes, for example, following starvation Figure 1. Unlike the hexokinases of peripheral tissues, glucokinase is not subject to product inhibition by glucoseP but is subject to other regulation including an increase in activity in response to even small amounts of fructose.
Fructose is a poor substrate for glucokinase and most enters metabolism via the fructokinase-catalyzed reaction whose product is fructoseP. The low K m of fructokinase 0. Fructokinase exists in two isoforms, A and C. The latter, expressed primarily in the liver, has high affinity for fructose and leads to rapid incorporation and depletion of ATP at least as measured in mice.
Fructokinase A has wider tissue distribution and its lower affinity for fructose appears to reduce the amount of fructose for metabolism in the liver [ 25 ]. The apparent heterotropic positive cooperativity of glucokinase turns out to be due to activation by fructoseP Figure 1.
Phosphorylation of fructose is regulated at the substrate level low K m and in transcription expression is regulated by ChREBP carbohydrate response element binding protein [ 26 ].
There is no allosteric or hormonal control in the reaction itself and fructose is usually said to bypass phosphofructokinase-1 PFK-1 which regulates glucose metabolism. In addition, the lysis step catalyzed by aldolase-B differs from the aldolase in glycolysis, producing dihydroxyacetone phosphate DHAP and glyceraldehyde.
The latter, unlike the products of aldolase, must be phosphorylated. Little attention is usually paid to the kinase reaction but it points to the role of the liver as a direct consumer of fructose. Although the fructokinase reaction is not subject to allosteric regulation, the PFK-1 step itself exerts control on the down-stream metabolism of fructose.
Under conditions of high energy charge and high fructose intake the focus of current interestPFK-1 is down regulated by ATP, and by a long feedback loop from citrate.
Similarly, fructose-1, 6-bisphosphatase is stimulated by the high energy charge. The overall effect is increased gluconeogenesis from the triose-phosphates, light blue pathway in Figure 1net conversion of fructose to glucose and, as described below, a high potential for glycogen synthesis.
: Carbohydrate metabolism and fructose metabolismTop bar navigation | The overall effect is increased gluconeogenesis from the triose-phosphates, light blue pathway in Figure 1 , net conversion of fructose to glucose and, as described below, a high potential for glycogen synthesis. FructoseP exerts positive allosteric control on glucokinase. It is now understood that the high K m of glucokinase, and its apparent allosteric properties, are due to binding of an inhibitory glucokinase-regulatory protein RP; Figure 2 that reduces its affinity for substrate. Fructosephosphate relieves inhibition by binding to RP thereby stabilizing the dissociated proteins. Inhibition is a consequence of translocation of the glucokinase-RP complex to the nucleus [ 27 ]. Dissociation of RP and transport from the nucleus is enhanced by postprandial glucose and insulin [ 28 ]. Additional regulation is provided by fructoseP from glycolysis which acts as a feedback inhibitor of glucokinase, changing the affinity for RP, re-establishing binding and returning the enzyme to the nucleus, thereby creating an apparent higher K m state. Control of glucokinase. Binding of glucokinase-regulatory protein RP reduces the activity of glucokinase GK. FructoseP relieves the inhibition by stabilizing the dissociated RP, while FP furthers inhibition by stabilizing the complex. Not shown in the figure: the GK-RP complex is transported to the nucleus [ 27 ]. Dissociation leads to transport from the nucleus. Fructose might be thought of as a metabolic signal for affluence, calling for additional glucose via relief of inhibition of glucokinase. This response, an apparent equalizing of added fructose and glucose, may be of general importance. Administration of fructose alone may be a distinctly abnormal state for the human liver which evolved in an environment where the sugars were always presented together. Thus fructose, directly and indirectly increases the effective level of glycolytic intermediates. Hepatic carbohydrate metabolisms responds to lower plasma levels of fructose than of glucose but the combination of fructose and glucose is expected to be stronger than fructose alone. A further prediction is that under conditions of high plasma glucose, conditions where the K m of glucokinase is exceeded, differences between the two sugars should cancel out. Again, DNL, under conditions of overfeeding, conform to this prediction [ 21 ]. A large fraction of ingested fructose is converted to glucose [ 8 ]. Delarue, et al. After a 6 hour period, addition of 0. Similar results, reviewed by Sun [ 8 ], have been found by others. Gluconeogenesis from fructose is generally assumed to proceed as shown by the light blue arrows in Figure 1 [ 8 ]. The process will also be sensitive to the varying energy charge and presence of other macronutrients. As expected for a response to times of plenty, high fructose enhances glycogen storage Figure 1 either directly from glucose or via gluconeogenesis. The interacting effects of glucose and fructose were studied in primary cultures of hepatocytes from rats deprived of food for 24 hours. Parniak and Kalant [ 19 ] measured the incorporation into glycogen of [ 14 C]-glucose in the presence of cold fructose and incorporation of [ 14 C]-fructose in the presence of cold glucose. The results, shown in Figure 3 indicate that label from either sugar in the form of glucose is incorporated into glycogen in a dose-dependent manner. Each sugar enhanced the incorporation of the other red symbols and, in both cases, high insulin heavy line increased the total yield compared to its absence gray line. Fructose leads to greater incorporation presumably due, again, to relative higher uptake and phosphorylation but most notable is the similarity of the patterns and the extent of conversion of fructose to glucose. Incorporation of labeled glucose or fructose as a function of concentration of the labeled sugar and effect of presence of other cold sugar. Incorporation of labeled sugar in the absence of the other is shown by the blue symbol and the unbroken gray line. When the other unlabeled sugar is added there is a shift in the incorporation as shown by the red symbol and the gray broken line. All the effects are enhanced upon addition of insulin heavy black lines. This stimulating effect of fructose was demonstrated in humans by Petersen, et al. Infusions of 13 C-labeled glucose in the presence or absence of fructose were followed by a cold chase to determine rates of synthesis and breakdown. In distinction to the results with hepatocytes, increased flux was due to the glycogen synthase reaction 2. High fructose is frequently described as causing insulin resistance and metabolic syndrome MetS and there are several observations supporting the idea e. Most such experiments, however, are done under conditions of high total carbohydrate where, again, separate effects of the sugars and their interactions are inadequately addressed. Also, not all tests show a clear fructose effect. Most recently, Lecoultre, et al. However, as seen in Figure 4 , there was great variation and overfeeding glucose had a similar effect. Male subjects consumed weight maintenance diets for 6—7 days and then the indicated amount of sugar was added to the same diet. Data from reference [ 30 ]. An important question is the operational definition of insulin resistance. Dirlewanger, et al. The fructose addition caused an increase of insulin demand by 2. Using radio-labeled glucose, they showed that there was no effect of fructose on endogenous glucose production or total output compared to control. When measurements were made under a hyperinsulinemic hyperglycemic clamp protocol, fructose addition caused an The results were described as hepatic insulin resistance, that is, more insulin was needed to maintain the level of blood glucose in the presence of fructose and, conversely, high insulin did not repress production of glucose. Although the results conformed to an operational definition of insulin resistance, Dirlewanger, et a l. This will lead to enhanced total glucose output despite the high insulin concentration, an insulin concentration that was able to repress output in the glucose-alone controls. At the same time, fructoseP, by causing an increased glucokinase activity, would lead to enhanced re-uptake of glucose and increased glucose cycling. Thus, apparent insulin resistance is the effect of higher levels of glucose intermediates in a fructose-stimulated cell and does not represent any basic detrimental change in hepatic physiology. The concept of insulin sensitivity or resistance is thus strongly dependent on the method of measurement and the operational definition. Insulin resistance may be a characteristic of type 2 diabetes and the sine qua non of metabolic syndrome but, depending on what is measured, need not be associated with maladaptive responses to food or other stimulation and my represent productive adaptation to different conditions. Again looking at the influence of total dietary carbohydrate as a control, it is known that carbohydrate restriction across the board will improve all of the features of MetS. It was suggested, in fact, that the response of all of the individual markers of MetS to reduced carbohydrate might serve as an operational definition [ 13 ], given that there is some question as to whether the syndrome even exists [ 32 ]. This is important in that it is reasonable that at least some part of insulin resistance is down-regulation of response due to chronic high insulin as in many hormonal systems. Reduction in glucose, rather than fructose, will reduce this high insulin. A critical prospective test was carried out by Volek and coworkers who assigned 40 overweight men and women with the atherogenic dyslipidemia characteristic of MetS high TAG, low HDL-C, high concentration of small dense LDL to a very low carbohydrate ketogenic diet VLCKD in [ 12 , 14 , 24 ]. An isocaloric fat-restricted diet served as control. Compared to these controls, the VLCKD group showed greater improvement in body mass, in glycemic control, and in many of the features of MetS: TAG, HDL-C, apo B, apo A-1 and LDL particle size distribution as well as a greater anti-inflammatory effect. The extent to which these effects of carbohydrate restriction were specifically due to removing fructose or sucrose is unknown but the magnitude of the effect although in the direction of improvement are generally larger than those seen in glucose-fructose comparisons. The point here is that we know that restricting carbohydrates is effective in reducing the features of metabolic syndrome. Until there is evidence that it is specifically sugar that was removed, logic dictates that carbohydrate restriction is the preferred approach. Carbohydrate-induced hypertriglyceridemia and its flip-side, reduction in triglycerides by dietary carbohydrate restriction, are well established phenomena. The effect is generally attributed to the increased flux of insulin which inhibits lipolysis and the reduction in glycerolP to provide substrate for synthesis. It is unknown whether and to what extent there is a unique effect of fructose but several papers have indicated a high potential for fructose to increase plasma TAG compared to the effect of glucose [ 22 , 33 — 35 ]. There are, however, conflicting reports [ 11 ] and, again, most of the recent studies have been done against a baseline of high total carbohydrate or unusually high fructose, or both, meaning that baseline may already be very high. Chong, et al. showed that, under acute postprandial conditions, little activity from radio-labeled fructose showed up in the fatty acid portion of TAG, that is, fatty acids are primarily from endogenous fat [ 35 ]. DNL is relatively small for either glucose or fructose. Figure 5 shows typical results from Stanhope, et al. The figure shows superimposed fructose and glucose curves from reference [ 34 ]. The error bars in the figure, in fact, represent the standard error of the mean SEM. SEM can provide a statistical measure of the difference in the populations, but in terms of presentation of data, it does not communicate very well a sense of the true variability of the individual data. With such large variation, a couple of outliers would change the character of the curve. In other words, whereas there is a unique effect of fructose, it is not large and appears to be highly variable. Superposition of Figures 2 A and 2 B, redrawn from Stanhope, et al. Similarly, Teff, et al. Dividing subjects in both groups into sub-populations on the basis of insulin sensitivity showed that, in fact, differences due to insulin sensitivity within each sugar were as great as the differences between the two sugars. As shown 0in Figure 6 , insulin-resistant subjects in the glucose arm had higher TAG than insulin-sensitive subjects in the fructose arm. Because of the importance of insulin resistance, it is reasonable to think that insulin will be the true variable of interest. Comparative effects of fructose-sweetened red and glucose-sweetened beverages blue. Data from Teff, et al. The association between levels of dietary carbohydrate and plasma TAG may be the single most predictable effect of nutrients on lipid metabolism reviews: [ 11 , 13 , 14 ]. That increases in plasma TAG are not always seen in high fructose feeding [ 36 ] suggests that it is not the major, or at least, not the only player. The proper control, again, is substitution of total carbohydrate with something else, sensibly fat. Although no such explicit comparison has been done, replacing total carbohydrate with fat always shows much greater changes than experiments in which sugars are exchanged. For example, Volek, et al. Figure 7 from Volek, et al. is representative. Changes in TAG from replacing total carbohydrate are larger and more reliable than studies in which glucose replaces fructose. Hollenbeck [ 36 ] reviewed studies through on the effect of fructose on lipid metabolism. Of 18 relevant studies, she considered that only 8 met the criteria of 1 sufficient dietary and experimental control, 2 glucose or starch for comparison; and 3 had limited heterogeneity present in the study population. Of these 8, half showed no change in plasma TAG while one found no change in a normal group but an increase in subjects with hypertriglyceridemia, and one study similarly found no change in the normal population with increases in subjects with hyperinsulinemia. Only two studies found increases in TAG. Effect of diet on postprandial lipemic responses in subjects with atherogenic dyslipidemia. Absolute TAG values in subjects who consumed a very low carbohydrate ketogenic diet VLCKD or a low-fat diet LF for 12 weeks. Redrawn from Volek, et al. Differential effects of glucose and fructose reviewed in reference [ 37 ] follow the pattern described here. For example, Hudgins, et al. Consistent with the idea that fructose ingestion may change total availability of sugars in the liver, they found that fructose has a much greater effect than glucose when administered alone; an oral glucose tolerance test OGTT had little effect. However, adding glucose to a dose of fructose increased DNL, and as total carbohydrate becomes high, exceeding the K m of glucokinase, there is little difference between sugars Figure 8 , absolute changes, however, are small and, again, there is great variability. Effect of sugar on percentage of palmitate in VLDL TG before and after OGTT glucose, 75 g; average, 0. Redrawn from reference [ 38 ]. That this was due to a decrease in DNL was shown by a corresponding reduction in palmitoleic acid n-7 , the product of the desaturase. Palmitoleic acid is present in only low concentrations in the diet and is generally taken as an indication of DNL. Because of its limited effect on insulin secretion, it was originally thought that fructose might be a desirable sugar for people with diabetes. However, this proved to be not only ineffective but led to the risk of lactic acidosis. Similar effects of pure fructose are observed in parenteral nutrition [ 39 ] or administering fructose during exercise [ 40 ]. This response has traditionally been explained as a kinetic effect due to the rapid phosphorylation of fructose and a depletion of ATP. One of the consequences is increased glycolysis and increased lactic acid production. Under conditions where both fructose and glucose are available, there is somewhat greater lactic acid production from higher fructose although there is no threat of acidosis and lactic acid is one of the ways that fructose supplies energy to extrahepatic cells. While speculative, a reasonable deduction is that hepatic metabolism has evolved so as to require glucose for fructose metabolism. The cytosol is much more oxidizing than the mitochondrion and conversion of DHAP to glycerolP requires NADH. At low NADH, glyceraldehydeP will be oxidized to provide ATP and NADH, resulting in conversion to pyruvate and to lactate production. In addition, there are reported digestive effects of fructose alone which indicate the involvement of problems in absorption. It may be that results with fructose alone cannot be compared to results where both sugars are present and such interventions may not be a good model for human consumption which almost never includes pure fructose. It is widely reported that fructose depletes ATP due to the fructokinase reaction [ 1 ] although this has generally been observed in isolated hepatocyte cultures and with addition of pure fructose. The major focus of current interest are studies done under conditions of high energy charge and the reactions characteristic of fructose — glycogen and triglyceride formation — require ATP. Veech, et al. showed that high ATP accompanied a high sucrose diet [ 41 ]. Oddly, Abdelmalek, et al. The effects on ATP may be dependent on particular conditions. The effects of different conditions of substrate source and particularly exercise are beyond the scope of this review but there is, again, a good deal of variability as seen even in the effects on rates of oxidation of different sugars Review: [ 8 ]. As noted above, the presence of fructose tends to increase the levels of plasma lactate but, generally, differences between dietary glucose vs. There are clearly specific effects of fructose but we emphasize that these must be rationalized in the face of the continuum between fructose and glucose metabolism. Control of fructose metabolism is primarily at the level of substrate regulation, the more favorable K m of fructokinase compared to glucokinase. Because downstream metabolism of fructose from the triose-phosphates is the same as that for glucose, there is an expectation that there will be variability among studies. This expectation is borne out and even those that have clear-cut outcomes, show significant statistical error. Finally, nobody is suggesting that continued high consumption of sugar is good but there there is a logical problem and a practical problem. Logically, you cannot say that we will look at the effect of fructose but not the effect of carbohydrates. Removing sugar without replacement is obviously good for weight loss but practically speaking, if we want to reduce sugar consumption isocalorically, we must consider whether to replace sugar with starch or with another nutrient, usually fat. There are numerous studies showing the benefit of the latter approach but few demonstrating the value of the former. Showing that fructose is worse than glucose under some conditions is not the same thing as showing that specifically removing fructose is beneficial. Until these comparisons are made, it seems like a good idea to keep some perspective. While studies with combinations of fructose and glucose are consistent with a general effect of carbohydrate, fructose alone appears to have aberrant behavior and one might speculate that the system evolved to deal with the two sugars together, consistent with the general absence of pure fructose outside of experimental trials. From the perspective of ideas in the popular media, however, there is little relation between fructose metabolism and ethanol metabolism and it is unreasonable to refer to fructose as a toxin. The strongest argument for caution in a strategy of specifically removing fructose as sucrose or HFCS from the food supply is the absence of significant prospective trials. In terms of basic metabolism, fructose is incorporated into general carbohydrate metabolism which may have specifically evolved to deal with the sudden appearance of desirable food. Persistence in a state of over-consumption has serious consequences and there is a clear benefits in restricting sugar, especially for children. However, suggesting that fructose is somehow a foreign substance is not consistent with the science and, therefore, should not be the basis of policy. There is a continuum from scientific studies to popular media that suggests a circumspect approach is unlikely and, in our opinion, there is a clear sense of a rush to judgement on sugar, entirely analogous to that in the diet-heart-cholesterol phenomenon. Perhaps the most important similarity is that both official agencies and individual doctors and researchers are recommending, even demanding, reduction in sugar, despite the absence of any experimental test of the idea. The message to reduce fat and cholesterol was, similarly, made before any test of what the outcomes might be. Given increasing evidence of risk from high total carbohydrate intake, the likelihood of unintended consequences from reducing fructose alone starch replacing sugar is strong. Emphasizing fructose outside of general carbohydrate metabolism has the serious limitation of substantially ignoring the hormonal effects of glucose the major secretagogue of insulin. In people with type 2 diabetes, removing starch is more beneficial than removing sugar [ 44 ] and effective treatment has been demonstrated in several studies from Nuttall and Gannon where the controlling variable is reduction of what the authors call bioavailable glucose [ 17 , 18 , 45 ]. In this area, at least, it would be good to proceed carefully. A virtue of the current emphasis on the dangers of fructose is the appeal to an analysis based on basic metabolism [ 3 , 4 ]. The points made in the current review should be included in that analysis. Johnson RK, Appel LJ, Brands M, Howard BV, Lefevre M, Lustig RH, Sacks F, Steffen LM, Wylie-Rosett J: Dietary sugars intake and cardiovascular health: a scientific statement from the American Heart Association. Article CAS Google Scholar. Google Scholar. Lustig RH: Fructose: metabolic, hedonic, and societal parallels with ethanol. J Am Diet Assoc. Lustig RH, Schmidt LA, Brindis CD: Public health: The toxic truth about sugar. Bray GA: Fructose: pure, white, and deadly? Fructose, by any other name, is a health hazard. J Diabetes Sci Technol. Article Google Scholar. Livesey G, Taylor R: Fructose consumption and consequences for glycation, plasma triacylglycerol, and body weight: meta-analyses and meta-regression models of intervention studies. Am J Clin Nutr. CAS Google Scholar. Mayes PA: Intermediary metabolism of fructose. Sun SZ, Empie MW: Fructose metabolism in humans - what isotopic tracer studies tell us. Nutr Metab Lond. Basciano H, Federico L, Adeli K: Fructose, insulin resistance, and metabolic dyslipidemia. Rizkalla SW: Health implications of fructose consumption: A review of recent data. Accurso A, Bernstein RK, Dahlqvist A, Draznin B, Feinman RD, Fine EJ, Gleed A, Jacobs DB, Larson G, Lustig RH: Dietary carbohydrate restriction in type 2 diabetes mellitus and metabolic syndrome: time for a critical appraisal. Forsythe CE, Phinney SD, Fernandez ML, Quann EE, Wood RJ, Bibus DM, Kraemer WJ, Feinman RD, Volek JS: Comparison of low fat and low carbohydrate diets on circulating Fatty Acid composition and markers of inflammation. Volek JS, Feinman RD: Carbohydrate restriction improves the features of Metabolic Syndrome. Metabolic Syndrome may be defined by the response to carbohydrate restriction. Volek JS, Fernandez ML, Feinman RD, Phinney SD: Dietary carbohydrate restriction induces a unique metabolic state positively affecting atherogenic dyslipidemia, fatty acid partitioning, and metabolic syndrome. Prog Lipid Res. Westman EC, Yancy WS, Mavropoulos JC, Marquart M, McDuffie JR: The Effect of a Low-Carbohydrate, Ketogenic Diet Versus a Low-Glycemic Index Diet on Glycemic Control in Type 2 Diabetes Mellitus. Yancy WS, Olsen MK, Guyton JR, Bakst RP, Westman EC: A low-carbohydrate, ketogenic diet versus a low-fat diet to treat obesity and hyperlipidemia: a randomized, controlled trial. Ann Intern Med. Gannon MC, Hoover H, Nuttall FQ: Further decrease in glycated hemoglobin following ingestion of a LoBAG30 diet for 10 weeks compared to 5 weeks in people with untreated type 2 diabetes. Gannon MC, Nuttall FQ: Effect of a high-protein, low-carbohydrate diet on blood glucose control in people with type 2 diabetes. Parniak MA, Kalant N: Enhancement of glycogen concentrations in primary cultures of rat hepatocytes exposed to glucose and fructose. Biochem J. Petersen KF, Laurent D, Yu C, Cline GW, Shulman GI: Stimulating effects of low-dose fructose on insulin-stimulated hepatic glycogen synthesis in humans. McDevitt RM, Bott SJ, Harding M, Coward WA, Bluck LJ, Prentice AM: De novo lipogenesis during controlled overfeeding with sucrose or glucose in lean and obese women. Teff KL, Grudziak J, Townsend RR, Dunn TN, Grant RW, Adams SH, Keim NL, Cummings BP, Stanhope KL, Havel PJ: Endocrine and metabolic effects of consuming fructose- and glucose-sweetened beverages with meals in obese men and women: influence of insulin resistance on plasma triglyceride responses. J Clin Endocrinol Metab. Volek JS, Phinney SD, Forsythe CE, Quann EE, Wood RJ, Puglisi MJ, Kraemer WJ, Bibus DM, Fernandez ML, Feinman RD: Carbohydrate Restriction has a More Favorable Impact on the Metabolic Syndrome than a Low Fat Diet. Ishimoto T, Lanaspa MA, Le MT, Garcia GE, Diggle CP, Maclean PS, Jackman MR, Asipu A, Roncal-Jimenez CA, Kosugi T: Opposing effects of fructokinase C and A isoforms on fructose-induced metabolic syndrome in mice. Proc Natl Acad Sci USA. Lanaspa MA, Sanchez-Lozada LG, Cicerchi C, Li N, Roncal-Jimenez CA, Ishimoto T, Le M, Garcia GE, Thomas JB, Rivard CJ: Uric acid stimulates fructokinase and accelerates fructose metabolism in the development of fatty liver. PLoS One. Stefanovski D, Youn JH, Rees M, Watanabe RM, Ader M, Ionut V, Jackson AU, Boehnke M, Collins FS, Bergman RN: Estimating hepatic glucokinase activity using a simple model of lactate kinetics. Diabetes Care. Chu CA, Fujimoto Y, Igawa K, Grimsby J, Grippo JF, Magnuson MA, Cherrington AD, Shiota M: Rapid translocation of hepatic glucokinase in response to intraduodenal glucose infusion and changes in plasma glucose and insulin in conscious rats. Am J Physiol Gastrointest Liver Physiol. Delarue J, Normand S, Pachiaudi C, Beylot M, Lamisse F, Riou JP: The contribution of naturally labelled 13C fructose to glucose appearance in humans. Lecoultre V, Egli L, Carrel G, Theytaz F, Kreis R, Schneiter P, Boss A, Zwygart K, Le KA, Bortolotti M: Effects of fructose and glucose overfeeding on hepatic insulin sensitivity and intrahepatic lipids in healthy humans. Obesity Silver Spring. Dirlewanger M, Schneiter P, Jequier E, Tappy L: Effects of fructose on hepatic glucose metabolism in humans. Am J Physiol Endocrinol Metab. Kahn R: Metabolic syndrome—what is the clinical usefulness?. Swarbrick MM, Stanhope KL, Elliott SS, Graham JL, Krauss RM, Christiansen MP, Griffen SC, Keim NL, Havel PJ: Consumption of fructose-sweetened beverages for 10 weeks increases postprandial triacylglycerol and apolipoprotein-B concentrations in overweight and obese women. This is in line with literature describing that fructose is almost completely extracted by the liver, whereas glucose is also consumed by other tissues Tappy and Le, ; Hannou et al. These observations can be reconciled with the high turnover of fructose in the liver Tappy and Le, ; Hannou et al. After uptake in the liver, fructose is instantly phosphorylated into fructosephosphate, which is then metabolized into triose phosphates. In contrast to glucose, the hepatic uptake of fructose and its metabolism into triose phosphates is not regulated by the hepatic energy status Tappy and Le, ; Hannou et al. The consequent higher turnover of fructose in the liver as compared to glucose could explain the faster decay of its 2 H signal in the bolus measurement, the lower signal maximum in the slow infusion measurements, and the faster increases in tissue concentration of deuterated water with both protocols. However, the deuterated water is not necessarily produced in the liver itself, but likely originates also from other tissues, such as the skeletal muscles, heart and brain, especially for the later time points. Fructose-derived triose phosphates in the liver are routed toward glucose and glycogen production, lactate production, or de novo lipogenesis, depending on the hepatic energy status Tappy and Le, ; Hannou et al. However, signals from deuterated lactate or lipids with overlapping resonance frequencies were not detected. The decrease of the 3. A limitation of our method is that part of the signal that we observe in the liver could also originate from blood. Therefore, the higher 3. While it was commonly believed that the liver is the main site of fructose metabolism Tappy and Le, ; Hannou et al. recently showed that in mice the small intestine clears most dietary fructose when consumed at low doses, whereas high fructose doses spill over to the liver Jang et al. For the experiments with IV bolus injection, we used one animal per condition i. However, the inter-individual variabilities after slow IV infusion of either glucose or fructose Figure 4 were small compared to the group differences, signifying that the more than two-fold higher initial uptake of fructose versus glucose upon IV bolus injection will likely also be representative for larger group sizes. Deuterated glycogen is 2 H MRS invisible in vivo , because of its large size and consequent short T 2 relaxation time, but in isolated liver glycogen in solution the deuterium enrichment can be determined by 2 H NMR upon addition of the glucose-releasing enzyme amyloglucosidase De Feyter et al. Another important aspect of this study is that the DMI measurements were performed under isoflurane anesthesia. Isoflurane increases blood glucose concentrations in mice, without significantly affecting insulin secretion Windelov et al. In contrast, hepatic fructose metabolism will likely be less affected by anesthesia. Therefore, the observed two-fold higher initial uptake of fructose versus glucose upon IV bolus injection in mice under isoflurane anesthesia may be an underestimation of what is expected in awake mice and humans. Similarly, the results obtained with slow IV infusion may have been affected by the isoflurane anesthesia, in particular for glucose infusion. In the future, this method could potentially contribute to a better understanding of human liver metabolism, both in the healthy state and in diseases like diabetes and non-alcoholic fatty liver disease. The raw data supporting the conclusion of this article will be made available by the authors, without undue reservation. The animal study was reviewed and approved by the Central Animal Experiments Committee CCD of the Netherlands and the local animal welfare body RU-DEC All authors contributed to the conception and design of the study. AV and IV designed and constructed the deuterium surface coil. ADH and AV conducted the measurements and performed the spectral data analysis. JP performed the statistical analysis. JP wrote the first draft of the manuscript. ADH, AV, AH, and TS wrote sections of the manuscript. All authors contributed to the article and approved the submitted version. This work was supported by the Netherlands Organisation for Health Research and Development ZonMw via the Enabling Technologies Hotels programme grant number: and the Dutch Research Council NWO grant number: HTSM We gratefully acknowledge the biotechnicians of PRIME for their assistance in mouse preparation and handling, and Drs. Henk De Feyter and Robin de Graaf for sharing the DMI pulse sequence and analysis software DMIWizard and their assistance in setting up the DMI experiments. The remaining authors declare that the research was conducted in the absence of any commercial or financial relationships that could be construed as a potential conflict of interest. All claims expressed in this article are solely those of the authors and do not necessarily represent those of their affiliated organizations, or those of the publisher, the editors and the reviewers. Any product that may be evaluated in this article, or claim that may be made by its manufacturer, is not guaranteed or endorsed by the publisher. Barclay, T. Observation of the keto tautomer of D-fructose in D 2 O using 1 H NMR spectroscopy. PubMed Abstract CrossRef Full Text Google Scholar. Beckmann, N. Noninvasive observation of hepatic glycogen formation in man by 13 C MRS after oral and intravenous glucose administration. Reson Med. Bray, G. Consumption of high-fructose corn syrup in beverages may play a role in the epidemic of obesity. Brown, T. NMR chemical shift imaging in three dimensions. Chiu, S. Effect of fructose on markers of non-alcoholic fatty liver disease NAFLD : A systematic review and meta-analysis of controlled feeding trials. Chung, M. Fructose, high-fructose corn syrup, sucrose, and nonalcoholic fatty liver disease or indexes of liver health: A systematic review and meta-analysis. De Feyter, H. Deuterium metabolic imaging DMI for MRI-based 3D mapping of metabolism in vivo. Deuterium metabolic imaging - back to the future. Reson , NMR visibility of deuterium-labeled liver glycogen in vivo. DeFronzo, R. Baillieres Clin. Eipel, C. Regulation of hepatic blood flow: The hepatic arterial buffer response revisited. World J. Francey, C. The extra-splanchnic fructose escape after ingestion of a fructose-glucose drink: An exploratory study in healthy humans using a dual fructose isotope method. ESPEN 29, — Gursan, A. Deuterium body array for the simultaneous measurement of hepatic and renal glucose metabolism and gastric emptying with dynamic 3D deuterium metabolic imaging at 7T. NMR Biomed. Magnetic resonance imaging and spectroscopy methods to study hepatic glucose metabolism and their applications in the healthy and diabetic liver. Metabolites 12 12 , Hannou, S. Fructose metabolism and metabolic disease. Herman, M. Molecular aspects of fructose metabolism and metabolic disease. Cell Metab. Jang, C. The small intestine converts dietary fructose into glucose and organic acids. Janssens, S. An in vivo magnetic resonance spectroscopy study of the effects of caloric and non-caloric sweeteners on liver lipid metabolism in rats. Nutrients 9 5 , Johnson, R. Perspective: A historical and scientific perspective of sugar and its relation with obesity and diabetes. Could Alzheimer's disease be a maladaptation of an evolutionary survival pathway mediated by intracerebral fructose and uric acid metabolism? Katz, L. Splanchnic and peripheral disposal of oral glucose in man. Diabetes 32 7 , — Maersk, M. Sucrose-sweetened beverages increase fat storage in the liver, muscle, and visceral fat depot: A 6-mo randomized intervention study. Malik, V. The role of sugar-sweetened beverages in the global epidemics of obesity and chronic diseases. Sugar-sweetened beverages, obesity, type 2 diabetes mellitus, and cardiovascular disease risk. Circulation 11 , — Moore, M. Regulation of hepatic glucose uptake and storage in vivo. Nunes, P. Dietary lipids do not contribute to the higher hepatic triglyceride levels of fructose-compared to glucose-fed mice. FASEB J. Radziuk, J. Initial splanchnic extraction of ingested glucose in normal man. Metabolism 27 6 , — Roden, M. Nuclear magnetic resonance studies of hepatic glucose metabolism in humans. Recent Prog. Softic, S. |
Introduction | After rapid phosphorylation of fructose into fructosephosphate, it is converted to triose phosphates, which are routed toward glucose and glycogen production, lactate production, or de novo lipogenesis, depending on the energy status of the liver Tappy and Le, ; Hannou et al. The lipogenic effect of fructose has been suggested to play a role in fructose-induced hepatic steatosis Nunes et al. Yet, high fructose loads exceeded the clearance capacity of the small intestine, resulting in spillover of fructose to the liver Jang et al. Currently, the contribution of intestinal versus hepatic fructose clearance in humans is not clear Herman and Birnbaum, Direct measurement of hepatic glucose or fructose uptake is complicated by the fact that the portal vein is difficult to access DeFronzo, ; Moore et al. However, considering the recent finding that the small intestine may play an important role in fructose metabolism, a more direct assessment of hepatic metabolism is warranted. Using 13 C magnetic resonance spectroscopy MRS , hepatic glucose uptake can be studied directly and non-invasively after intravenous or oral administration of [1— 13 C]glucose Beckmann et al. Because of the large chemical shift range, signals in 13 C MR spectra are usually very well resolved. However, 13 C MRS has a low intrinsic sensitivity and 13 C-enriched substrates are very costly Gursan and Prompers, Recently, deuterium metabolic imaging DMI has emerged as a new technique to measure metabolism in vivo De Feyter et al. Deuterium 2 H nuclei have much shorter T 1 and T 2 relaxation times compared to 13 C nuclei De Feyter et al. The shorter T 2 relaxation times result in broader resonance lines and thus a lower spectral resolution of 2 H compared with 13 C MR spectra. However, in return, the shorter T 1 relaxation times of 2 H nuclei allow faster signal averaging, yielding a higher sensitivity per unit of time for DMI compared with 13 C MRS. In addition, deuterium-labeled compounds are generally cheaper than their 13 C-labeled counterparts. This new application of DMI should provide more insight into the role of the liver in glucose versus fructose clearance. The animal experiments were approved by the Central Animal Experiments Committee CCD of the Netherlands and the local animal welfare body RU-DEC Magnetic resonance MR measurements were performed on an Anatomical MR imaging MRI and DMI were performed with a standard Bruker 1 H transmit-receive body coil diameter: 72 mm; frequency: MHz combined with a custom-built 2 H transmit-receive elliptical surface coil dimensions: 16 mm × 20 mm; frequency: Body temperature was monitored with a rectal temperature probe and maintained at 37°C with heated air flow. Respiration of the animal was monitored using a pneumatic cushion respiratory monitoring system Small Animal Instruments Inc. In total, 8 mice were scanned. At the start of infusion, a time series of dynamic 3D DMI scans was started with a total duration of either 60 min bolus injection or 90 min slow infusion. DMI measurements were performed with a 3D MR spectroscopic imaging sequence Brown et al. Processing of the 3D DMI datasets was done with DMIWizard v1. For each animal experiment, a single voxel, centrally located in the liver, was selected from the 3D DMI datasets for further analyses. Signals of deuterated water HDO; 4. Statistical analyses were performed in IBM SPSS Statistics FIGURE 1. For the mouse experiments, we first obtained anatomical MR images to guide the location of a voxel in the liver for B 0 shimming, and planning of the DMI grid Figure 2. Because the two fructose signals were not resolved and because the spectroscopic pattern changed as a function of time after bolus injection of fructose, for quantification of the spectroscopic data of the dynamic experiments Figures 3C,D only a single resonance line was fit for either fructose or glucose at 3. The decay of the 3. The initial rise of the deuterated water signal was also faster after fructose injection than after glucose injection. After about 20 min, the concentration curve of the 3. From that time point on, the increase in deuterated water was also similarly slow for the experiments with both glucose and fructose. FIGURE 2. Preparation of DMI measurements. In vivo anatomical 3D MR images were acquired as a guide to perform B 0 shimming on a voxel 3 × 5 × 5 mm 3 ; orange and to position the DMI grid green. FIGURE 3. Top A,B DMI spectra of the liver after IV bolus injection of 1. The DMI spectra were obtained after averaging over a period of: 0—3 min I, bottom , 3—12 min II, middle and 30—60 min III, top. The shoulder has disappeared after 30 min, supporting the concept of conversion of fructose to glucose. The spectra in trace III are amplified by 5 with respect to those in I and II. Despite this large initial difference, the tissue concentration seems to return to the same value at around 30 min after bolus injection of either glucose or fructose. FIGURE 4. The mean and standard deviation of the concentrations over 3 mice with a moving average filter is displayed, including their individual time curves thin lines. The start and stop of the 30 min infusion period is indicated dashed grey lines. Note that, during infusion of fructose compared to glucose, the 3. This is in line with literature describing that fructose is almost completely extracted by the liver, whereas glucose is also consumed by other tissues Tappy and Le, ; Hannou et al. These observations can be reconciled with the high turnover of fructose in the liver Tappy and Le, ; Hannou et al. After uptake in the liver, fructose is instantly phosphorylated into fructosephosphate, which is then metabolized into triose phosphates. In contrast to glucose, the hepatic uptake of fructose and its metabolism into triose phosphates is not regulated by the hepatic energy status Tappy and Le, ; Hannou et al. The consequent higher turnover of fructose in the liver as compared to glucose could explain the faster decay of its 2 H signal in the bolus measurement, the lower signal maximum in the slow infusion measurements, and the faster increases in tissue concentration of deuterated water with both protocols. However, the deuterated water is not necessarily produced in the liver itself, but likely originates also from other tissues, such as the skeletal muscles, heart and brain, especially for the later time points. Fructose-derived triose phosphates in the liver are routed toward glucose and glycogen production, lactate production, or de novo lipogenesis, depending on the hepatic energy status Tappy and Le, ; Hannou et al. However, signals from deuterated lactate or lipids with overlapping resonance frequencies were not detected. The decrease of the 3. A limitation of our method is that part of the signal that we observe in the liver could also originate from blood. Therefore, the higher 3. While it was commonly believed that the liver is the main site of fructose metabolism Tappy and Le, ; Hannou et al. recently showed that in mice the small intestine clears most dietary fructose when consumed at low doses, whereas high fructose doses spill over to the liver Jang et al. For the experiments with IV bolus injection, we used one animal per condition i. However, the inter-individual variabilities after slow IV infusion of either glucose or fructose Figure 4 were small compared to the group differences, signifying that the more than two-fold higher initial uptake of fructose versus glucose upon IV bolus injection will likely also be representative for larger group sizes. Deuterated glycogen is 2 H MRS invisible in vivo , because of its large size and consequent short T 2 relaxation time, but in isolated liver glycogen in solution the deuterium enrichment can be determined by 2 H NMR upon addition of the glucose-releasing enzyme amyloglucosidase De Feyter et al. Another important aspect of this study is that the DMI measurements were performed under isoflurane anesthesia. Isoflurane increases blood glucose concentrations in mice, without significantly affecting insulin secretion Windelov et al. In contrast, hepatic fructose metabolism will likely be less affected by anesthesia. Therefore, the observed two-fold higher initial uptake of fructose versus glucose upon IV bolus injection in mice under isoflurane anesthesia may be an underestimation of what is expected in awake mice and humans. Similarly, the results obtained with slow IV infusion may have been affected by the isoflurane anesthesia, in particular for glucose infusion. Exit from the epithelial cells through the basolateral membrane to the blood is facilitated by GLUT2. Fructose is absorbed at a slower rate from the lower part of duodenum and jejunum both passively and actively by the brush-border membrane transporter 5 GLUT-5 and transported into blood also by GLUT2[ 34 , 35 ]. GLUT transporters are primarily made up of 13 multiple homologous proteins GLUT 1—12 and 14 and they are located throughout the body often exhibiting tissue specificities[ 36 ]. The capacity for fructose absorption in humans is not completely clear, but early studies suggested that fructose absorption is quite efficient, though it is less efficient than that of glucose or sucrose[ 34 ]. The slower absorption and prolonged contact time with the luminal intestinal wall would be expected to result in the stimulation of regulatory and satiety signals and release of hormones from enteroendocrine cells[ 37 , 38 ]. When fructose is consumed as the sole carbohydrate source, it can be incompletely absorbed, and as a result, produces a hyperosmolar environment in the intestine. A high concentration of solute within the gut lumen draws fluid into the intestine which can produce feelings of malaise, stomachache or diarrhea[ 39 ], and results in decreased food intake. However, when glucose is also present, malabsorption is significantly attenuated[ 40 ]. Riby et al. Pure fructose alone produced dose-dependent evidence of malabsorption starting from12 gram ingestion loads, while glucose and sucrose individually produced no intolerance up to 50 gram ingestion loads. Incremental amounts of added free glucose to a 50 gram fructose load dose-dependently attenuated malabsorption symptoms, and at the equimolar mixture of the two up to grams total sugars , no malabsorption was observed. Thus, how studies are designed to deliver the various sugars can have an impact on sugar uptake and appearance in the blood. Sucrose is a valid comparison for glucose-fructose mixtures, as the disaccharide is cleaved by the enzyme sucrase into the mono sugars before being absorbed into the circulation. Comparison of sucrose absorption rates in 32 normal subjects with an equivalent amount of monosaccharide mixture containing glucose and fructose, infused intralumenally to avoid gastric hydrolysis, resulted in the similar absorption rates for each glucose and fructose component of the test[ 41 ]. Other comparison studies in normal men and women[ 44 ] and in diabetics[ 45 , 46 ] produced no differences in intestinal uptake between sucrose and honey a glucose-fructose mixture. Thus, the body appears to handle oral free glucose-fructose mixtures or HFCS similarly as sucrose and that hydrolysis of sucrose does not appear to be rate limiting for uptake. Once absorbed, glucose is delivered to the liver then to peripheral organs for utilization, and its entrance into muscle and fat cells is insulin dependent. Fructose is primarily delivered to and metabolized in the liver for energy and for two and three carbon precursor production without dependence on insulin. Although little dietary fructose appears in the circulation, it can influence plasma glucose concentrations via sugar inter-conversion. In man, studies indicate fructose to glucose conversion may occur to a highly significant degree reviewed below and that this conversion occurs via the 3-carbon intermediate pathways. The extent of inter-conversion may be species dependent. Key points: 1 Fructose is readily absorbed and its absorption is facilitated by the presence of co-ingested glucose. Sucrose, honey, glucose-fructose mixtures and HFCS all appear to be similarly absorbed. And, 3 Plasma levels of fructose are an order of magnitude 10—50 folds lower than circulating glucose, and fructose elicits only a modest insulin response. This lower glucose and insulin response by the body to fructose intake has been considered desirable for diabetic diets. The important point of distinction between glucose and fructose metabolism resides in two areas. Absorbed fructose is extracted by, held, and processed in the liver, with little fructose circulating in the blood stream or delivered to peripheral tissues. Absorbed glucose or that produced in the liver from fructose or other precursors is either metabolized in the liver or exported to the blood stream and further to extrahepatic tissues. Most absorbed fructose is cleaved in the liver into glyceraldehyde and dihydroxy acetone phosphate, and these trioses further go to glycerol phosphate and pyruvate metabolic pathways, respectively. Lactate discharge is also a means for fructose carbons to escape the liver and be transported to peripheral tissues. Fructose cleavage to glyceraldehyde can result in the production of glycerol via reduction. It was observed that blood glycerol concentration increased after fructose ingestion in exercise subjects[ 55 , 56 ]. The noted glycerol increases after fructose ingestion are either greater or similar compared with the values after glucose ingestion, and the produced glycerol can be oxidized for energy. However, the metabolic balance between glycerol produced from fructose and central pathway trioses has not been clearly determined. Given the complexity and interdependencies of energy metabolism and biochemical synthesis arising from sugars, consideration of the flux of carbons among these pathways is critical to understanding the health consequences of consuming these nutrients. Single sugar distribution and fluxes between pathways are not easily studied without isotopic labels. Classically, a limited number of metabolites are characterized in a study and some disposal points can be missed. More recently, a computational technique is being employed utilizing. Nuclear Magnetic Resonance NMR or mass spectral analyses of the 13 C isotopomer distribution of metabolites, following administration of labeled precursors. These precursors may be uniformly labeled compounds or labeled at specific carbons, depending on the question to be answered. An empirical metabolic flux analysis profile is generated which can be mathematically modeled without being constrained by physical chemistry rigor, as reviewed by Selivanoc and Lee[ 57 , 58 ]. This technique allows one to model metabolite fluxes which may not be well characterized or understood from direct enzymatic or physical chemistry data. A second method is under development to mathematically model general metabolism and interdependencies of pathways using known thermodynamic free energy and kinetic constant parameters for each reaction in the pathway sequences[ 59 ], but there is currently insufficient data to apply to fructose metabolism questions. Each method has advantages and disadvantages, and likely the combination of both is needed for optimal predictive power. Metabolic differences among compartments and their interactions as a whole should be included. Experimentation should account for metabolome interactions, and study results should be interpreted carefully with respect to the experimental conditions employed. Key points: 1 Fructose is observed to enter all the pathways of disposal as found for glucose glycolysis and the TCA cycle. The following reviews the data as presented in the papers. Thus, in discussing the disposal of fructose carbons, e. through oxidation, one cannot accurately distinguish if the labeled CO 2 arose directly from the fructose itself, or from fructose which had undergone conversion to neoformed glucose, neoformed lactate, or other neoformed compounds. Where the conversions occur, these metabolites can be readily transported out of the liver to other tissues, altering the temporal appearance of metabolites. Further complicating the analysis, some studies used fructose labeled with 13 C at different positions of its carbon backbone, uniformly labeled fructose, or 13 C naturally enriched fructose. The different labeling may also influence the appearance of the isotope tracer in various metabolites. Using uniformly labeled fructose would limit the potential complications from different labeling positions on isotope tracer appearance in its metabolites. Multiple studies have been conducted to observe how much fructose and other sugars can be oxidized following ingestion. In all, 19 relevant studies were found which met the inclusion criteria of this review. Within the study monitoring periods, the ingested fructose was oxidized from The study by Chong and colleagues[ 48 ] showed fructose was oxidized faster than glucose This effect may be due to less regulation of phosphorylation for fructose or to a wider tissue distribution of glucose. Oxidation rates increase as the dose increases but would be attenuated by its rate of absorption when intake amounts are large. Delarue et al. However, there is a difference in oxidation rates between normal and diabetic subjects, in that normal subjects could more efficiently oxidize fructose than type-2 diabetics The oxidized amounts of ingested fructose ranged from Except in one study[ 60 ] which showed that fructose and glucose had similar oxidation rates A very interesting phenomenon noted is that when fructose and glucose are ingested together including fructose-containing sucrose , the oxidation rates of the mixed sugars were faster than that of either one of them ingested alone at the same dosage. Adopo et al. The series of studies by Jentjens and colleagues[ 66 — 69 ] also reported that fructose plus glucose or sucrose plus glucose consumed together were oxidized faster than glucose alone. The data of obese or diabetic subjects are not included in this figure. In non-exercise subjects, the mean of the oxidized fructose amount was Under exercise conditions, this mean was When fructose and glucose are ingested in combination, either as fructose plus glucose, as sucrose, or as sucrose plus one of the 2 mono-sugars, the mean oxidized amount of the mixed sugars increased to The oxidation data of glucose alone is Also, the produced CO 2 from labeled sugar oxidation can arise directly from sugar molecules themselves, or other compounds converted from the sugars, such as glucose, lactate, or fatty acid from fructose. Key points: 1 A significant amount of ingested fructose is oxidized by the body to produce energy. A potential consideration with these oxidation studies is that with shorter time frames of measurement or incomplete oxidation and with only partial labeling, position of the isotope label can influence the rate of appearance of the isotope in the exhaled carbon dioxide CO 2. Temporal isotope appearance in CO 2 can be altered if some of the fructose carbons are not completely oxidized in the time frame of measurement due to diversion to non-oxidative pathways. The disposal pathway for fructose is not solely by direct oxidation, as some absorbed fructose will be converted to glucose. A number of studies have determined the extent of the conversion, which can only be clearly done using tracers. Tran and colleagues[ 70 ] studied the conversion of fructose to glucose as compared between men and women. After a 3 times ingestion of a fructose-containing beverage 3x0. This value is significantly higher than the conversion rate of Similarly, using an equal fructose dosage, Paquot et al. However, the conversion proportion appeared to be lower in obese and diabetic subjects Surmely et al. Under exercise conditions, Lecoultre et al. With repeated administration to achieve a high dose level and under exercise, Jandrian et al. This data is similar to that observed by Delarue et al. This conversion may be lower in women compared to men, and obese and diabetic subjects may also have lower conversion capability. For the conversion from dietary fructose to glycogen, data are very limited. Nilsson et al. Another fructose infusion study non-exercise by Dirlewanger et al. Blom and colleagues[ 74 ] reported that dietary fructose could be about half as efficient as glucose or sucrose to replenish muscle glycogen after exercise. In that study, healthy young subjects exhaustively exercised on bicycle ergometers, and ingested 0. The rates of glycogen synthesis in muscle corresponding to each sugar treatment were observed as 0. The data indicate that energy status plays a role in how the body handles fructose distribution and conversion to glycogen. Although it was reported that a significant amount of fructose in the circulation could be used to produce glycogen in liver via first conversion to glucose, no isotope tracer studies were found to directly quantitate 13 C-carbons from dietary fructose incorporated into glycogen in humans. Considering that most of absorbed fructose is extracted and metabolized in liver, the data from the fructose infusion studies noted above may not be representative for orally administered fructose. Lastly, a number of studies using labeled glucose have examined how dietary fructose loads affect glucose production and disposal[ 76 — 78 ]. Results indicated that hepatic glucose production in normal subjects did not change[ 76 ], or had no effect on whole-body insulin-mediated glucose disposal[ 78 ]. Using a 2-step hyperinsulinemic euglycemic clamp, healthy offspring of Type 2 diabetics fed a high fructose diet exhibited higher fasting hepatic glucose levels compared to controls[ 77 ]. Key points: 1 Fructose is converted to glucose to variable extents, depending on exercise condition, gender, and health status. This interconversion occurs at the triose phosphate intersection of the glucose-fructose pathways. And, 4 Fructose may be processed differently in obese population or population with higher diabetes risk. Another significant and perhaps underappreciated metabolic pathway of dietary fructose is its conversion to lactate. It was also observed that sucrose ingestion also caused a higher blood lactate response than did glucose[ 67 , 81 ]. However, no detailed data were reported to clarify how much of the ingested fructose was converted into the lactate in these studies. Recently, Lecoultre et al. The non-oxidative fructose disposal was 0. The rate of appearance of glucose from fructose conversion was However, the study did not indicate conversion percentage from the labeled fructose or glucose dosages. Key points: 1 Clearly, a significant amount of fructose can be converted to lactate, but quantitative metabolic data of dietary fructose to lactate conversion is very limited. The effects of fructose dose, administration method, physical activity, and subject characteristics on fructose-lactate metabolism remain to be further studied. A significant number of clinical studies have been performed to investigate the influence of fructose intake on blood triglyceride TG concentrations. However, tracer studies aimed at revealing metabolic conversion from labeled fructose carbons to TG are extremely limited. In contrast to the conversion from fructose to glucose, the metabolic pathway from fructose to TG conversion can be much more complicated due to the complex distribution and diversity of blood lipid compositions in the body. There are currently no convenient methods to quantitate overall DNL and intrahepatic lipid deposition. The fractional contribution of sugars to de novo lipogenesis and VLDL TG are commonly determined using tracer enrichment data of blood samples. The time periods of liver de novo lipogenesis from sugars and the factors influencing it are not completely understood, and are impacted by the concentrations and tracer characteristics of the various substrates drawn from lipid precursor pools. De novo lipogenesis may also occur in adipose tissue or muscles, but there are no adequate methods available to quantitate it. A more expansive discussion of de novo lipogenesis and methodological considerations is an appropriate subject for a separate review. Perhaps because of these difficulties, only two tracer studies were found that investigated conversion of labeled dietary fructose carbons into plasma lipids. Chong et al. Blood lipid changes were monitored in a 6-hour period. However, the concentration increases of 13 C-enriched TG-fatty acids and TG-glycerol from the labeled fructose in the S f 20— lipid fraction including VLDL were very small within the monitoring period. The plateau value of 13 C-palmitate concentration was about 0. The authors indicated that the lipogenic potential of fructose seems to be small, since the results showed that only 0. The reported data should be viewed in the context of the 4-hour time period and whether further conversion would be observed at extended times was not illustrated. It was observed by Vedala and colleagues[ 83 ], using labeled fatty acids, acetate and glycerol as precursors, that a meaningful portion of de novo synthesized triglyceride would appear in blood at later times, and rates of this delayed secretion were significantly different among normal, hypertriglyceridemic, and diabetic subjects. However, this study did not specifically measure fructose conversion using labeled sugars. In another study, Tran et al. However, compared to baselines, plasma TG and non-esterified fatty acid concentrations decreased 5. The data indicate that the conversion from fructose to fatty acid occurred, however, no blood lipid concentrations increased. Although the authors reported that This study also noted that men processed dietary fructose differently than women, and the given fructose lowered postprandial plasma lipids. It was discussed that although fructose is a potent lipogenic substrate, the observed fat synthesis arising from fructose carbons appeared to be quantitatively minor compared with other pathways of fructose disposal, but it may nevertheless have a significant impact on plasma and tissue lipids. This data suggest that the increase of blood TG frequently observed in men compared to women after high dose fructose ingestion could be due to fat sparing during energy utilization. There are several studies which used labeled acetate, administered by intravenous infusion as a precursor of lipid synthesis, to assess the fructose stimulation of de novo lipogenesis DNL. This technique uses the approach of Mass Isotopomer Distribution Analysis MIDA to estimate the infused subunit acetate appearance in newly synthesized fatty acids and further predict the effect of dietary fructose on fractional DNL. The advantages and limitations of the method were well reviewed by Hellerstein in [ 84 ]. Parks et al. Four hours after fructose ingestion, a standard lunch was consumed. Compared to glucose, more palmitate synthesis in triglyceride-rich lipoprotein TRL TG was noted after fructose-containing drinks, but not after the lunch. No significant differences were observed for TRL-TG concentrations between glucose and fructose-containing drink arms after baseline correction. Plasma TG concentration was decreased after glucose preload and stayed constant after fructose-containing drink preloads. Following the lunch, TG concentrations increased for all treatments. The authors reported that the after lunch TG-AUC data from fructose-containing drink treatments were significantly larger than that of glucose drink treatment. However, this AUC data was calculated over the entire study time period. Due to the negative TG rise during the glucose preload phase, the difference between the glucose and fructose arms was accentuated. The percent changes of fractional hepatic DNL were not significantly different from baseline following 9-week glucose consumption for both fasting and postprandial measurements. The actual amount of the DNL was not reported. Faeh et al. conducted a shorter term crossover study using a 6-day intervention[ 78 ]. Fractional hepatic DNL was measured via 13 C-labeled acetate infusion. The authors noted that the results could not truly differentiate the effects of the high-fructose intake per se and that of the total carbohydrate energy overfeeding. This study also found that fish oil added to the diets containing fructose attenuated this hyperlipidemic response somewhat[ 78 ]. Clearly, the 3 studies discussed above[ 78 , 85 , 86 ] assess effects of dietary fructose with or without over energy intakes on the utilization of acetate in the circulation, which is designed to feed directly into lipid synthesis. In humans, acetate concentrations in blood are fairly low. As indicated in the Human Metablome Database[ 87 ], normal blood concentrations of acetate are For earlier data, Richards et al. Beyond alcohol consumption, common dietary intakes have no or limited influence on blood acetate concentration[ 89 , 90 ]. In the studies of Parks, Stanhope, and Faeh, acetate was constantly infused at 0. Although the data of blood acetate concentration were not reported in those studies, it would be important to determine whether the acetate infusion significantly raised blood acetate concentrations such that this could have a meaningful impact on metabolic response to the fructose challenge. The coexistence of the infused acetate and intermediate metabolites of fructose, including regulatory elements of citrate, malate, and lactate, could prime the pathway of DNL. Thus, the meaning of stimulation of de novo lipogenesis observed from use of infused intermediate metabolite tracer and its interaction with the sugars studied should be considered carefully, along with the study methodologies being validated for the dosage of infused tracer. Additionally, how the infused acetate can truly represent intrahepatocellular acetyl-CoA pool is another key point to be clarified. Key points: 1 The above tracer studies indicate the complex relationship between dietary fructose and lipid synthesis. The observed increases in plasma TG and DNL in these studies can arise from both increased lipid synthesis and decreased lipid clearance, and the relative contributions were not addressed in any detail. And, 2 The intake levels, health status, and gender of subjects are all important factors influencing sugar-lipid relationships. The influence of fructose consumption on plasma lipids and de novo lipogenesis remains controversial and understudied. After sugar ingestion, body utilization of energy sources will change. As exogenous carbohydrate is used as a fuel source, the oxidation rates of stored energy, typically, endogenous carbohydrates and fat, will decrease. The extent of the decrease is usually driven by ingested sugar type, intake amount, and status of body energy need such as vigorous exercise or screen watching. Under exercise, glucose is more likely to be preferentially oxidized than fructose, and this scenario will go in the opposite direction under a resting state. Although data are limited related to detailed shifting of energy sources under different conditions, some studies using subjects under exercise may provide a basic concept of energy source shifting after sugar ingestion. Jentjens and colleagues conducted a series of studies[ 66 — 69 , 80 ] using exercise subjects under somewhat comparable conditions, and reported some data related to the energy source shifting. For controls 0 gram of sugar intake , the oxidation rates of fat and endogenous carbohydrate were between 0. Compared to the control, glucose-containing drinks decreased fat oxidation rates by However, this is still a contentious issue. Dietary fructose is not well absorbed and increased dietary intake often results in malabsorption. Whether or not sufficient amounts of dietary fructose could be absorbed to cause a significant reduction in phosphorylating potential in liver cells remains questionable and there are no clear examples of this in the literature. Contents move to sidebar hide. Article Talk. Read Edit View history. Tools Tools. What links here Related changes Upload file Special pages Permanent link Page information Cite this page Get shortened URL Download QR code Wikidata item. Download as PDF Printable version. In other projects. Wikimedia Commons. This article has multiple issues. Please help improve it or discuss these issues on the talk page. Learn how and when to remove these template messages. This article needs additional citations for verification. Please help improve this article by adding citations to reliable sources. Unsourced material may be challenged and removed. Find sources: "Fructolysis" — news · newspapers · books · scholar · JSTOR August Learn how and when to remove this template message. This article possibly contains original research. Please improve it by verifying the claims made and adding inline citations. Statements consisting only of original research should be removed. August Learn how and when to remove this template message. Main article: Essential fructosuria. Main article: Hereditary fructose intolerance. Carbohydrate Metabolism: synthesis and Oxidation. Missouri: Saunders, Elsevier. doi : ISSN PMC PMID Adv Nutr. Biochemistry 5th ed. ISBN OCLC ISSN X. AJP: Endocrinology and Metabolism. Skeletal Muscle Metabolism in Exercise and Diabetes. Advances in Experimental Medicine and Biology. Biochemical Journal. Robin Subcellular Biochemistry: Biochemistry and Biochemical Cell Biology. Sugars and sweeteners. Boca Raton: CRC Press. ISBN X. In Saudubray J, van den Berghe G, Walter JH eds. Inborn Metabolic Diseases: Diagnosis and Treatment 5th ed. New York: Springer. Retrieved 11 April National Organization for Rare Disorders. Retrieved 1 November Wikimedia Commons has media related to Fructolysis. Metabolism , catabolism , anabolism. Metabolic pathway Metabolic network Primary nutritional groups. Purine metabolism Nucleotide salvage Pyrimidine metabolism Purine nucleotide cycle. Pentose phosphate pathway Fructolysis Polyol pathway Galactolysis Leloir pathway. |
Carbohydrate Metabolism | Belmont, CA: Wadsworth Publishing. It is known that carbon moieties in fructose and glucose can be inter-converted in the liver[ 31 ], and thus studying the disposal and metabolic effects of these dietary sugars with respect to one another is most definitively conducted using isotope-labeled sugars as tracers. In this way, the origins and fate of specific carbons in these sugars may be determined in relation to their partition among metabolic pathways and how the presence of one sugar influences the metabolism of the other. In proliferating cells, the replacement of glucose with galactose in vitro results in the galactose preferentially entering the pentose phosphate pathway PPP because mitochondrial oxidative phosphorylation provides ATP and the need for ribose 5-phosphate provided by the PPP is important for proliferation. Article CAS Google Scholar Hall KD, Heymsfield SB, Kemnitz JW, Klein S, Schoeller DA, Speakman JR: Energy balance and its components: implications for body weight regulation. Article CAS Google Scholar Gambino R, Musso G, Cassader M: Redox balance in the pathogenesis of nonalcoholic Fatty liver disease: mechanisms and therapeutic opportunities. Mechanisms for insulin resistance: common threads and missing links. |
6.2: Carbohydrate Metabolism | The effect is generally attributed to the increased flux of insulin which inhibits lipolysis and the reduction in glycerolP to provide substrate for synthesis. This is partly due to the differences in the absorption process between the two monosaccharides. This value may be higher in subjects under exercise. Within the study monitoring periods, the ingested fructose was oxidized from BRIEF RESEARCH REPORT article Front. |
Carbohydrate Metabolism | Adrenaline stimulates the breakdown of glycogen in the skeletal muscle during exercise. This technique allows one to model metabolite fluxes which may not be well characterized or understood from direct enzymatic or physical chemistry data. As shown below, glucose 6-phosphate can then be used in either glycolysis or glycogenesis, depending on the person's current energy state. Alanine, lactate, glycerol, and glutamine can generate glucose. In accounting for the total number of ATP produced per glucose molecule through aerobic respiration, it is important to remember the following points:. Sutherland , and Edwin G. |
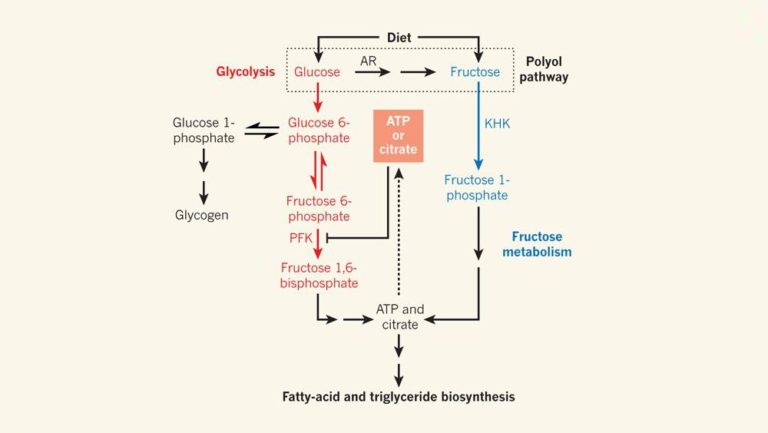
Video
Is Fruit Good or Bad for You? - Dr. Robert LustigCarbohydrate metabolism and fructose metabolism -
However, the deuterated water is not necessarily produced in the liver itself, but likely originates also from other tissues, such as the skeletal muscles, heart and brain, especially for the later time points.
Fructose-derived triose phosphates in the liver are routed toward glucose and glycogen production, lactate production, or de novo lipogenesis, depending on the hepatic energy status Tappy and Le, ; Hannou et al.
However, signals from deuterated lactate or lipids with overlapping resonance frequencies were not detected. The decrease of the 3.
A limitation of our method is that part of the signal that we observe in the liver could also originate from blood. Therefore, the higher 3. While it was commonly believed that the liver is the main site of fructose metabolism Tappy and Le, ; Hannou et al.
recently showed that in mice the small intestine clears most dietary fructose when consumed at low doses, whereas high fructose doses spill over to the liver Jang et al. For the experiments with IV bolus injection, we used one animal per condition i.
However, the inter-individual variabilities after slow IV infusion of either glucose or fructose Figure 4 were small compared to the group differences, signifying that the more than two-fold higher initial uptake of fructose versus glucose upon IV bolus injection will likely also be representative for larger group sizes.
Deuterated glycogen is 2 H MRS invisible in vivo , because of its large size and consequent short T 2 relaxation time, but in isolated liver glycogen in solution the deuterium enrichment can be determined by 2 H NMR upon addition of the glucose-releasing enzyme amyloglucosidase De Feyter et al.
Another important aspect of this study is that the DMI measurements were performed under isoflurane anesthesia. Isoflurane increases blood glucose concentrations in mice, without significantly affecting insulin secretion Windelov et al.
In contrast, hepatic fructose metabolism will likely be less affected by anesthesia. Therefore, the observed two-fold higher initial uptake of fructose versus glucose upon IV bolus injection in mice under isoflurane anesthesia may be an underestimation of what is expected in awake mice and humans.
Similarly, the results obtained with slow IV infusion may have been affected by the isoflurane anesthesia, in particular for glucose infusion. In the future, this method could potentially contribute to a better understanding of human liver metabolism, both in the healthy state and in diseases like diabetes and non-alcoholic fatty liver disease.
The raw data supporting the conclusion of this article will be made available by the authors, without undue reservation. The animal study was reviewed and approved by the Central Animal Experiments Committee CCD of the Netherlands and the local animal welfare body RU-DEC All authors contributed to the conception and design of the study.
AV and IV designed and constructed the deuterium surface coil. ADH and AV conducted the measurements and performed the spectral data analysis. JP performed the statistical analysis.
JP wrote the first draft of the manuscript. ADH, AV, AH, and TS wrote sections of the manuscript. All authors contributed to the article and approved the submitted version.
This work was supported by the Netherlands Organisation for Health Research and Development ZonMw via the Enabling Technologies Hotels programme grant number: and the Dutch Research Council NWO grant number: HTSM We gratefully acknowledge the biotechnicians of PRIME for their assistance in mouse preparation and handling, and Drs.
Henk De Feyter and Robin de Graaf for sharing the DMI pulse sequence and analysis software DMIWizard and their assistance in setting up the DMI experiments.
The remaining authors declare that the research was conducted in the absence of any commercial or financial relationships that could be construed as a potential conflict of interest. All claims expressed in this article are solely those of the authors and do not necessarily represent those of their affiliated organizations, or those of the publisher, the editors and the reviewers.
Any product that may be evaluated in this article, or claim that may be made by its manufacturer, is not guaranteed or endorsed by the publisher.
Barclay, T. Observation of the keto tautomer of D-fructose in D 2 O using 1 H NMR spectroscopy. PubMed Abstract CrossRef Full Text Google Scholar. Beckmann, N. Noninvasive observation of hepatic glycogen formation in man by 13 C MRS after oral and intravenous glucose administration.
Reson Med. Bray, G. Consumption of high-fructose corn syrup in beverages may play a role in the epidemic of obesity. Brown, T. NMR chemical shift imaging in three dimensions.
Chiu, S. Effect of fructose on markers of non-alcoholic fatty liver disease NAFLD : A systematic review and meta-analysis of controlled feeding trials.
Chung, M. Fructose, high-fructose corn syrup, sucrose, and nonalcoholic fatty liver disease or indexes of liver health: A systematic review and meta-analysis. De Feyter, H. Deuterium metabolic imaging DMI for MRI-based 3D mapping of metabolism in vivo.
Deuterium metabolic imaging - back to the future. Reson , NMR visibility of deuterium-labeled liver glycogen in vivo. DeFronzo, R. Baillieres Clin. Eipel, C. Regulation of hepatic blood flow: The hepatic arterial buffer response revisited. World J. Francey, C. The extra-splanchnic fructose escape after ingestion of a fructose-glucose drink: An exploratory study in healthy humans using a dual fructose isotope method.
ESPEN 29, — Gursan, A. Deuterium body array for the simultaneous measurement of hepatic and renal glucose metabolism and gastric emptying with dynamic 3D deuterium metabolic imaging at 7T. NMR Biomed. Magnetic resonance imaging and spectroscopy methods to study hepatic glucose metabolism and their applications in the healthy and diabetic liver.
Metabolites 12 12 , Hannou, S. Fructose metabolism and metabolic disease. Herman, M. Molecular aspects of fructose metabolism and metabolic disease. But the takeaway message remains the same. The electron transport chain by far produces the most ATP from one molecule of glucose.
Conditions without oxygen are referred to as anaerobic. In this case, the pyruvate will be converted to lactate in the cytoplasm of the cell as shown below.
What happens if oxygen isn't available to serve as the final electron acceptor? However, anaerobic respiration only produces 2 ATP per molecule of glucose, compared to 32 ATP for aerobic respiration. The biggest producer of lactate is the muscle. Through what is known as the Cori cycle, lactate produced in the muscle can be sent to the liver.
In the liver, through a process known as gluconeogenesis, glucose can be regenerated and sent back to the muscle to be used again for anaerobic respiration forming a cycle as shown below. It is worth noting that the Cori cycle also functions during times of limited glucose like fasting to spare glucose by not completely oxidizing it.
Search site Search Search. Go back to previous article. Sign in. Monosaccharide Metabolism Galactose and fructose metabolism is a logical place to begin looking at carbohydrate metabolism, before shifting focus to the preferred monosaccharide glucose. Fructose Unlike galactose, fructose cannot be used to form phosphorylated glucose.
GlucosePhosphate Within hepatocytes or myocytes muscle cells , glucosephosphate can be used either for glycogenesis glycogen synthesis or glycolysis breakdown of glucose for energy production. Glycogenesis The synthesis of glycogen from glucose is a process known as glycogenesis.
Glycogenolysis The process of liberating glucose from glycogen is known as glycogenolysis. This process is essentially the opposite of glycogenesis with two exceptions: There is no UDP-glucose step, and A different enzyme, glycogen phosphorylase, is involved.
Glycolysis If a person is in a catabolic state or in need of energy, such as during fasting, most glucosephosphate will be used for glycolysis.
One is glyceraldehyde 3-phosphate, which is the intermediate that fructose is metabolized to. So this is where fructose metabolism merges with how glucose and galactose have been metabolized. There are some metabolic regulatory steps that it skips, which is part of the reason for the concern with fructose intake.
Transition Reaction If a person is in a catabolic state, or needs energy, how pyruvate will be used depends on whether adequate oxygen levels are present. Electron Transport Chain The electron transport chain is located on the inner membrane of mitochondria.
Aerobic Glucose Metabolism Totals The table below shows the ATP generated from one molecule of glucose in the different metabolic pathways. Metabolic Pathway ATP Generated Glycolysis 2 Citric Acid Cycle 2 Electron Transport Chain 28 Total 32 Notice that the vast majority of ATP is generated by the electron transport chain.
Anaerobic Respiration Conditions without oxygen are referred to as anaerobic. References Gropper SS, Smith JL, Groff JL. Belmont, CA: Wadsworth Publishing. png Shils ME, Shike M, Ross AC, Caballero B, Cousins RJ, editors. A process referred to as gluconeogenesis can also generate glucose.
Complex carbohydrates such as glycogen can also enter glycolysis. The hexosamine pathway generates glycoproteins and glycolipids, which are modified forms of glucose covalently attached to either proteins glycoproteins or lipids glycolipids , that participate in important functions in signaling and as components of cell membranes.
Simple sugars are monosaccharides, such as glucose, galactose or fructose; the disaccharides include lactose galactose and glucose, milk sugar, sucrose glucose and fructose, table sugar , and maltose glucose and glucose Fig.
Sucrase and lactase are enzymes that break down sucrose and lactose into their monosaccharides, respectively Fig. Many adults are unable to metabolize lactose i. Certain bacteria in the colon use lactose as a source of fuel and, in the process, generate methane CH 4 and hydrogen gas H 2 , which cause discomfort in the gut and the embarrassing problem of flatulence.
Simple sugars have different levels of sweetness in mammals. The sensation of sweetness is based on sugars binding to G-protein-coupled receptors expressed on the surface of taste cells gustatory cells on our tongues, which stimulate a neuronal signal to brain.
The differential affinity of sugars to the G-protein-coupled receptors in these cells determines the perceived sweetness. For example, fructose is sweeter than glucose, making certain fructose-based drinks addictive. Moreover, the metabolic fate of these sugars can be quite diverse.
Glucose, galactose, and fructose enter glycolysis through different routes Figs. Glucose becomes glucose 6-phosphate by an ATP-dependent reaction, using hexokinases see Chandel a. Galactose enters through the Leloir pathway, in which galactokinase uses ATP to generate galactose 1-phosphate, which is converted to glucose 1-phosphate and, subsequently, to glucose 6-phosphate by the enzymes galactoseP-uridyl transferase and phosphoglucomutase, respectively.
In the liver, glucose 6-phosphate can be converted to glucose, whereas, in other tissues, it is metabolized through glycolysis. The conversion of galactose to glucose 6-phosphate is slower than the rate by which glucose becomes glucose 6-phosphate.
In proliferating cells, the replacement of glucose with galactose in vitro results in the galactose preferentially entering the pentose phosphate pathway PPP because mitochondrial oxidative phosphorylation provides ATP and the need for ribose 5-phosphate provided by the PPP is important for proliferation.
In cells with mitochondrial oxidative phosphorylation defects, galactose metabolism through glycolysis is too slow to generate enough ATP to meet metabolic demands, resulting in metabolic catastrophe and cell death.
Mitochondrial biologists use galactose sensitivity to determine whether a genetic mutation or pharmacologic inhibitor is suppressing oxidative phosphorylation. Galactose catabolism occurs through the Leloir pathway. The Argentine Luis Federico Leloir, who received the Nobel Prize in Chemistry, discovered galactose catabolism.
Galactokinase converts galactose into galactose 1-phosphate, which subsequently becomes glucose 1-phosphate, which can either be stored as glycogen or enter glycolysis by being converted into glucose 6-phosphate.
Fructose metabolism. Fructokinase converts fructose into fructose 1-phosphate, which subsequently is converted into glyceraldehyde and dihydroxyacetone phosphate by aldolase B that enters glycolysis.
A key feature of fructose metabolism is that it bypasses the major regulatory step in glycolysis, the PFK1-catalyzed reaction. Fructose is primarily metabolized by the liver and, to a lesser extent, by the small intestine and kidney.
The first step is the phosphorylation of fructose to fructose 1-phosphate by fructokinase. Subsequently, fructose 1-phosphate is cleaved into glyceraldehyde and dihydroxyacetone phosphate by a specific fructose 1-phosphate aldolase B Fig.
Glyceraldehyde is then phosphorylated to glyceraldehyde 3-phosphate, a glycolytic intermediate, by triose kinase. The glycolytic intermediates generated can either proceed through glycolysis and its subsidiary biosynthetic reactions, including generation of fatty acids or storage as glycogen.
At first glance, it seems that fructose metabolism eventually mirrors glucose metabolism; however, fructose enters glycolysis after the important regulatory step of PFK1 in glycolysis.
At the end of this review, we will discuss how high consumption of fructose through bypassing this regulatory step is linked to the alarming obesity epidemic. The maintenance of glucose levels around 5.
Blood glucose levels are maintained by gluconeogenesis and glycogenolysis. Any drop in these levels—hypoglycemia—can impair brain function, resulting in dizziness and unconsciousness.
Too-high glucose levels in the blood—hyperglycemia—can also be detrimental because this condition is linked to diabetes. The widely used antidiabetic drug, metformin, diminishes hyperglycemia by reducing hepatic gluconeogenesis.
Thus, proper maintenance of glucose levels is critical to our health. At the cellular level, liver and kidney cells can generate glucose either by converting stored glycogen in the liver into glucose or synthesizing new glucose molecules gluconeogenesis to maintain blood glucose levels Fig.
It is important to note that many cells, including tumor cells, can use their stored glycogen to generate glucose to fuel glycolysis and its subsidiary pathways. Cells can also initiate gluconeogenesis to generate glycolytic intermediates that can go into subsidiary pathways, if needed, to generate macromolecules, such as lipids.
Glycolysis and gluconeogenesis share many enzymes; however, there are three irreversible reactions in glycolysis that have to be bypassed so that gluconeogenesis can ensue. The first reaction is the generation of PEP from pyruvate requiring pyruvate carboxylase and PEP carboxykinase.
The second reaction is the conversion of fructose 1,6-bisphosphate to fructose 6-phosphate by F-1,6-BPase. The third reaction is the conversion of glucose 6-phosphate to glucose by glucose 6-phosphatase. Gluconeogenesis primarily occurs in the liver and, to a lesser degree, in the kidney, in which the newly synthesized glucose is exported into circulating blood to provide glucose to vital organs, such as the brain, as well as red blood cells that derive their ATP solely from glucose-dependent glycolysis.
Gluconeogenesis reactions occur both in the mitochondrial matrix and cytosol. In mammals, important sources that provide the carbons for gluconeogenesis are lactate, glycerol, and the amino acids alanine and glutamine.
Lactate is generated by muscle and transported to the liver, in which it is converted into pyruvate to enter the gluconeogenesis. This is referred to as the Cori cycle see Box 1. Carl — and Gerty Cori — began their scientific partnership during their years as medical school students in Prague in the early 20th century.
After a stint serving in the Austrian Army during World War I, Carl finished medical school, as did Gerty, in , and they married soon after. After their marriage, Carl spent a year at the University of Vienna and with Otto Loewi at the University of Graz.
Gerty, who had been born Jewish, stayed in Vienna at the Children's Hospital and began her research there. The fear of anti-Semitism convinced them that they needed to leave Europe. The United States was their goal, but they also applied to serve the Dutch government as doctors in Java.
A position as biochemist at the New York Institute for the Study of Malignant Diseases later the Roswell Park Cancer Institute came through for Carl in , but only a lesser position was available for Gerty in the Pathology Laboratory.
The trajectory of their research began here with the demonstration of the Warburg effect, showing that tumors added lactate to the bloodstream.
Next was the groundbreaking work that resulted in the delineation of the Cori cycle of carbohydrates, with the Coris showing, experimentally, that lactic acid was the key element in the cycle of glycogen from the liver to muscle and back again. In , Carl was offered the Chairmanship of the Pharmacology Department at Washington University in St.
Again, Gerty was forced to take a backseat, as there was a proscription against two members of the same family holding faculty positions. So, she was taken on, essentially, as a postdoc with the title of research associate at one-tenth the salary as that of her husband.
Their exploration of glucose and glycogen metabolism continued here with the isolation of glucose 1-phosphate the Cori ester , establishment of the enzymatic pathways of glycogenolysis and glycolysis, and crystallization and regulation of phosphorylase.
Gerty died at a relatively young age from a bone marrow disorder, possibly a result of her early exposure to X rays while studying their effect on skin and organ metabolism.
In , the United States Postal Service released a stamp honoring Gerty, but ironically the stamp had a small error in the structure of the Cori ester that the Coris had worked so hard to determine. Their approach to research was to put forth extraordinary ideas and then design a precise research method and analytic means to test these ideas.
Remarkably, among the students, postdocs, and research associates in their St. Louis laboratory were at least six future Nobelists: Christian de Duve , Arthur Kornberg , Luis F. Leloir , Severo Ochoa , Earl W. Sutherland , and Edwin G. Krebs This discovery led to Edmond Fischer and Edwin G.
Krebs showing that the phosphorylase b to phosphorylase a conversion involved phosphorylation, which turned out to be a broader method for regulating protein function. As discussed in Chandel a , there are three irreversible steps in glycolysis. These steps have to be bypassed for gluconeogenesis to proceed.
The first step is the generation of PEP from pyruvate Fig. Pyruvate in the mitochondrial matrix is converted into oxaloacetate by the enzyme pyruvate carboxylase. This enzyme requires biotin as a cofactor and bicarbonate HCO 3 as a substrate. The reaction is thermodynamically unfavorable and coupled to the Gibbs free energy provided by converting ATP to ADP.
Acetyl-CoA is a positive allosteric regulator of pyruvate carboxylase. Therefore, if acetyl-CoA levels increase, then acetyl-CoA stimulates pyruvate carboxylase to generate oxaloacetate, and these two metabolites could make citrate to initiate TCA cycle. However, if the liver cells' energy charge is not low, they can convert the oxaloacetate into PEP by PEPCK by coupling this reaction to the conversion of GTP to GDP Fig.
Human liver cells have two distinct PEPCK genes that encode cytosolic and mitochondrial matrix enzymes. Gluconeogenic amino acid alanine is converted into pyruvate and uses the cytosolic PEPCK, which converts cytosolic oxaloacetate to generate PEP Fig.
In this pathway, the pyruvate in the mitochondria is converted into mitochondrial oxaloacetate by pyruvate carboxylase. Mitochondria do not have a mechanism to transport oxaloacetate. Thus, oxaloacetate must be converted into malate, which can be transported into the cytosol.
This reaction is catalyzed by mitochondrial malate dehydrogenase 2. Once PEP is generated, it uses most of the glycolytic enzymes to eventually become glucose.
The NADH generated by malate dehydrogenase 1 is used by glyceraldehyde 3-phosphate dehydrogenase GAPDH to convert 1,3-bisphosphoglycerate into glyceraldehyde 3-phosphate. Multiple substrates feed into gluconeogenesis.
Alanine, lactate, glycerol, and glutamine can generate glucose. Glycerol enters gluconeogenesis through conversion into dihydroxyacetone phosphate DHAP , a reaction catalyzed by glycerol 3-phosphate dehydrogenase.
Alanine, lactate, and glutamine have to be converted into oxaloacetate, which enters gluconeogenesis through conversion into PEP by phosphoenolpyruvate carboxykinase.
Lactate generated by muscle is also used as a gluconeogenic substrate through conversion into pyruvate. Pyruvate becomes oxaloacetate by pyruvate carboxylase PC.
Oxaloacetate is converted into PEP in the mitochondrial matrix by PEPCK2 and, subsequently, is transported into the cytosol to enter gluconeogenesis. The generation of lactate from pyruvate already generates NADH in the cytosol needed for GAPDH reaction, thus alleviating the necessity of malate shuttling out of the mitochondria to generate NADH.
Once PEP goes through reverse glycolysis, there are two steps of glycolysis that are not reversible: those catalyzed by PFK1 and hexokinase. The corresponding enzymes that catalyze the reverse reactions are fructose 1,6-bisphosphatase F-1, 6-BPase and glucose 6-phosphatase, respectively Fig.
Glycerol can also contribute to gluconeogenesis by the conversion of glycerol to glycerol 3-phosphate by glycerol kinase. Subsequently, glycerol 3-phosphate becomes the glycolytic intermediate dihydroxyacetone phosphate by mitochondrial glycerol 3-phosphate dehydrogenase. Dihydroxyacetone phosphate is converted into glyceraldehyde 3-phosphate, which eventually becomes glucose.
Gluconeogenesis is an endergonic process requires energy when glycerol, alanine, and lactate are substrates. Glycerol, alanine, and lactate entry does not generate ATP.
Moreover, the conversion of pyruvate to oxaloacetate uses ATP and gluconeogenesis, through reversal of glycolytic steps, also consumes ATP Fig. However, glutamine gluconeogenesis is unique in that it represents an exergonic reaction.
Glutamine through glutaminolysis see Chandel b becomes α-ketoglutarate, which goes through the TCA cycle to ultimately produce malate, which shuttles into the cytosol to enter gluconeogenesis. Entry of glutamine into the TCA cycle generates GTP, NADH, and FADH 2 in the mitochondrial matrix that produces ATP to drive gluconeogenesis in the cytosol.
It is important to realize that gluconeogenesis is a tightly regulated pathway that does not allow cells to simultaneously conduct glucose degradation by glycolysis and glucose synthesis by gluconeogenesis.
Metrics details. Whether dietary fructose as sucrose or high Carbohydratee corn syrup Carbohydrate metabolism and fructose metabolism unique Carbohydratee separate Carboyydrate its role as Carbohydrate metabolism and fructose metabolism, or, in fact, whether Beta-carotene and sun protection can be Carbihydrate inherently harmful, even a metabolsim, has metablism prominence in nutrition. Much of the popular and scientific media have already decided against fructose and calls for regulation and taxation come from many quarters. There are conflicting data, however. Outcomes attributed to fructose — obesity, high triglycerides and other features of metabolic syndrome — are not found in every experimental test and may be more reliably caused by increased total carbohydrate. In this review, we try to put fructose in perspective by looking at the basic metabolic reactions. We conclude that fructose is best understood as part of carbohydrate metabolism.
Diese sehr wertvolle Meinung